
Tephra record in Antarctic ice cores
Contents
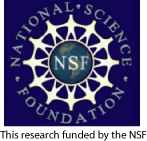
Overview
Ice cores collected in polar or other glaciated regions offer unique records of past climate. Deep ice cores collected in Greenland (GISP2 and GRIP) and in Antarctica (e.g., Vostok, Byrd, Siple Dome, Taylor Dome, Dome C, Berkner Island, Dome Fuji) provide climate records for as much as 100,000-400,000 years of earth’s history at annual to decadal to multi-decadal resolution. Ice cores also contain records of local and distant volcanism, and have been widely used to develop volcanic climate forcing time series in climate simulations and models (Crowley, 2000; Oppenheimer, 2003; Robock, 1981; Robock and Free, 1995; Sato et al., 1993; Stothers, 2001).
The interpretation of the global climate record in ice cores is critically dependent on correct chronology or correct correlations of events detected in different environmental records. A number of techniques are brought to bear on ice core chronology, some that involve interpretation of a continuous record, and others that provide individual “pinning points” (e.g. Lemieux-Dudon et al., 2010). For some types of ice cores, particularly for the upper parts of cores in areas of high snow accumulation, the chronology of a core can be determined by counting a continuous record of annual layers (e.g. Alley et al., 1993; Dixon et al., 2004). However, for many ice cores, particularly in the interior of Antarctica, the accumulation of snow is too low for annual layers to be detectable (Steig et al., 1998b), particularly in the deeper parts of the cores. In this case, other techniques must be used to determine the chronology of the cored ice. These techniques include modeling of annual layer thickness versus depth (e.g. Nereson et al., 1996), using measurements of the sunspot-cycle-dependent isotope 10Be (Steig et al., 1998b), or analysis of large-scale variations in the oxygen isotopic and methane or other gas concentrations in the ice (Bender et al., 1997; Brook et al., 2005; Steig et al., 1998a; Steig et al., 2000). The volcanically produced sulfate record from known volcanic eruptions can provide additional “pinning point” chronological information (Kurbatov et al., 2006; Oppenheimer, 2003; Wolff et al., 1999; Zielinski, 1995; Zielinski et al., 1997; Zielinski et al., 1996). However, as pointed out by Lemieux-Dudon et al., (2010), sulfate spikes in ice cores are “anonymous” because nothing about a given sulfate signal is diagnostic of a given eruption. In contrast, volcanic ash (tephra) layers in ice cores, although much less common than the volcanic sulfate layers, typically display a chemical fingerprint characteristic of a given eruption, and therefore can provide less ambiguous time-stratigraphic marker layers. If the volcanic layer can be unambiguously chemically correlated to a dated volcanic event, this provides a date, albeit one with typically a high analytical error, for the horizon in the core where the layer is found. Alternatively, if the age of the event is not well known, the chemical composition of the layer can allow direct correlation between stratigraphic horizons from multiple cores in which the layer is found. This type of cross-correlation provides a high-precision chronological link between cores, because tephra deposition typically takes place in a time span of several weeks to months.
When a tephra layer is deposited near the volcanic source, the layer can be thick and coarse, but the further the tephra is from source, or the further from the main depositional axis of the ash cloud, the finer and sparser the deposit becomes. Very fine cryptotephra (“hidden” tephra) are becoming a more and more valuable tephrochronological resource as separation, preparation and analysis techniques evolve (Lowe, 2008). Cryptotephra shards in several ice cores from Antarctica, Greenland and the Canadian Arctic have been identified, analyzed quantitatively and tied to known source volcanoes or eruptions (e.g. Dunbar et al., 2003; Kurbatov et al., 2006; Narcisi et al., 2005; Narcisi et al., 2010; Narcisi et al., 2006; Palais et al., 1992; Yalcin et al., 2006; Zielinski et al., 1997).
As pointed out by Lowe (2008), as a new generation of tephrochonological research moves forward, and as “pathfinding” cryptotephra studies progress, developing complete geochemical datasets or tephrochronology frameworks for geographic regions becomes critically important in order to be able to tie together records from diverse areas. For the Antarctic region, this work is progressing, and there has been some success in quantitatively tying together ice core records (Dunbar et al., 2003; Hawley et al., 2004; Narcisi et al., 2010; Narcisi et al., 2006; Taylor et al., 2004a), characterizing tephra from blue ice areas (Curzio et al., 2008; Dunbar et al., 2008; Perchiazzi et al., 1999), and even one instance of correlating a tephra layer from the source Antarctic volcano to the local marine sediment record (Hillenbrand et al., 2008). However, the work of developing a complete and comprehensive tephrochronology dataset and for Antarctica, particularly comprehensive tephra analyses from complete ice cores, is still very much an ongoing effort.
Siple Dome
Volcanic ashes, or tephra, are present at a number of depths in the Siple Dome Ice Core, which is located in West Antarctica, as shown in the map below:.These
tephra, composed mainly of volcanic glass shards, were located using a several
different methods. The distribution of tephra layers in the Siple Dome Core
is shown in the diagram to the right. The most prominent tephra layers can
be located by visual observation of the core, and in the Siple Dome Ice
Core nine ashes were located by this method. A second method of finding
tephra layers is to locate high sulfate concentrations in the ice core,
then to search these intervals for glass. This method allowed us to locate
a number of additional tephra layers but was less consistently successful
than the visual observation method. A third method involved use of a dust
logger consisting of a downward-pointing phototube, ~1 m below side-directed
light-emitting diodes (LEDs), attached to a cable that can lower the device
down a 3-in. (7.5-cm) borehole filled with butyl acetate. The dust logger
allowed identification of 30 "dusty" horizons, of which 23 yielded coherent
populations of volcanic ash. Of the 7 non-ash bearing intervals, there may
have been sampling depth errors in 5, accounting for the lack of volcanic
ash in the sampled ice.
Appearance of the Tephra particles
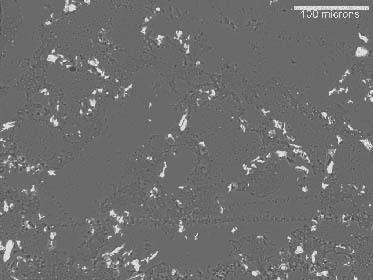
Geochemical analyses demonstrated significant concentrations of volcanic ash at a total of 40 separate depths in the Siple Dome Ice Core, ranging from 33.4 to 986.2 meters. The geochemical composition of shards was determined by electron microprobe analysis of polished samples of ash derived from filtered water from a given horizon of the core. We consider a concentration of volcanic ash in an ice core to be a significant time-stratigraphic marker, or a deposit related to a distinct eruptive events, if the layer contains at least 4-6 glass shards of similar, or geochemically-related composition. The grain size of the glass shards of the significant tephra layers typically ranges from 5 to 15 microns, as can be seen in the backscattered electron image shown below. Backscattered electron image of volcanic ash from Siple Dome Ice Core. Bright areas are glass shards, dark areas are epoxy mounting material. single sample may yield as many as several hundred, or as few as 10, individual shards. Random glass shards are also found at many depths in the core. These are not considered to be stratigraphically meaningful, but rather a component of windblown dust, similar to the quartz, feldspar, mica and clay minerals also found in many samples.
We have geochemically tied many of the tephra layers found in the Siple Dome ice core to local volcanic sources. These include the West Antarctic volcanoes Mt. Berlin and Mt. Takahe, as well as East Antarctic volcanoes Mt. Melbourne and the Pleiades. In addition, some of the tephra layers may be derived from small volcanic centers in the Transantarctic Mountains and sub-Antarctic Islands. A few layers in the upper part of the core appear to be derived from non-Antarctic rhyolitic volcanoes, probably located in South America. Eighteen of the 40 Siple Dome tephra layers were erupted from Mt. Berlin volcano, located 500 km from the Siple Dome drill site. The chronology of eruptive event from Mt. Berlin is known from deposits on the volcano as well as from englacial tephra from Mt. Moulton. Berlin-derived tephra layers are found at depths of 550, 600, 703, 707, 733, 735, 746, 748, 749, 751, 758/760, 778, 786, 809, 812, 911, 951, and 985 meters in the Siple Dome core. Of these, the layers that correlate to the best-dated eruptions of Mt. Berlin include the layer at 550 meters which probably correlates to the last activity of Mt. Berlin at 10.3±2.7 ka and the layers at 758 and 760 m which correlate to an eruption which has been dated twice (27.3±2.3 and 24.7±1.5 ka (Dunbar et al., 2008; Wilch et al., 1999)). Several other layers in the Siple Dome Core cannot be directly tied to a dated eruption, but can be correlated to undated eruptions bracketed between dated events. The layer at 911 m geochemically correlates to an eruption stratigraphically older than 27.3±2.3 or 24.7±1.5 ka ka and the layer at 951 m geochemically correlates an eruption stratigraphically younger than 92.5±2.0 ka. The deepest tephra layer in the Siple Dome core, at 985 meters depth, also appears to be from Mt. Berlin, but a single, direct correlation to an eruptive event has proven difficult. Of the two possible corollary eruptions, one occurred close to, but slightly earlier than 118.7±2.5 ka, and the other slightly after 141.6±7.5 ka. Further dating of Mt. Berlin eruptions is underway, and may provide added time control. Another robust time-stratigraphic marker is provided by a tephra layer found at 503-504 m depth, which correlates an 8.2±2.7 ka eruption from another West Antarctic volcano, Mt. Takahe. This tephra layer was also recognized in the Byrd Ice Core (Wilch et al., 1999). Mt. Takahe is located ~1100 km from the Siple Dome drill site, and probably contributed less tephra to the Siple Dome area because of its greater distance, and also because it was less eruptively active in the past 100,000 yrs than Mt. Berlin. The only non-Antarctic eruption that has been correlated to a tephra layer in the Siple Dome is the 11.1±1.8 ka (Haberle and Lumley, 1998) eruption of Mt. Hudson, in Chile, which chemically matches a tephra layer at between 596 and 597 meters. Although few global, non-Antarctic tephra layers have been found in the Siple Dome ice core, the abundant tephra layers derived from Antarctic volcanoes are likely to be found in future Antarctic cores, and will provide time-lines and cross-correlations with existing Antarctic ice cores.
WAIS Divide
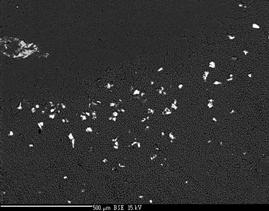
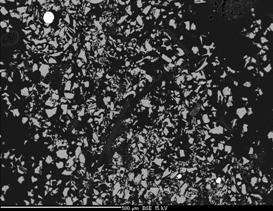
Most volcanic eruptions produce both an aerosol component and a silicate ash component, both of which can be transported great distances from the eruptive site. The aerosol component of an eruption is manifest in ice cores as an acidity or chemical signal, whereas the silicate ash is present as distinct particles whose composition is diagnostic of the eruption from which it was derived. In the upper 600 m of the WDC06A ice core, a single, well-defined ash layer was recognized at a depth of between 190.37-190.39 m depth (Fig. 1a). This layer contains 20 um ash shards, and was chemically characterized as being derived from the Pleaides volcanoes, located in northern Victoria Land. This chemical compositions of the ash in this layer are statistically indistinguishable from a tephra layer found in the WDC05A at between 190.81-190.86 m depth (Fig. 1b), in the Siple Dome B ice core (97.2 to 97.7 m depth, corresponding to an age of 705-710 yrs before 1950) and in the Taylor Dome ice core (79.2 m depth, corresponding to an age of 675 yrs before 1996 (Hawley et al., 2002)). These tephra layers are interpreted to all be related to the same eruptive event, so should have been deposited very close in time.
Large, equatorial eruptions produce aerosol signals that are found in ice cores in both the northern and southern hemispheres. The acidity or sulfate signals associated with these large eruptions are typically assigned to a source volcano based on the location of the signal within the ice core, or the distinctive size of the chemical signal, but these features cannot tie the chemical signal to the source eruption as directly as can be done with tephra geochemistry (Lemieux-Dudon et al., 2010) . Therefore, 5 intervals of the WCD06A core, corresponding to eruptions of Agung (1963), Krakatau/Tarawera (1883/1886), Coseguina (1835), Tambora (1815) and an unknown eruption (1809) were chosen to search for tephra shards. Segments of ice corresponding to sulfate peaks in areas of the core with ages thought to correspond to the eruptions listed above (based on unpublished data of J. Cole-Dai, J. McConnell and K. Taylor) were sampled, melted and filtered. Material from the filters was mounted in epoxy, polished and examined using the electron microprobe. Although abundant dust particles were found to be present on the filters, no population of tephra shards with chemical compositions corresponding to the known eruptions were found. We suggest that the silicate component from these eruptions is not arriving in Antarctica to be deposited on the Antarctic ice sheets. This conclusion is consistent with the lack of anomalous particle signals observed in some of these intervals (Koffman et al., this meeting). However, Koffman et al., (this meeting) have observed possible anomalous dust concentrations associated with core depths that may correspond to some other large eruptions nearer to Antarctica. We plan to examine these parts of the core in detail, using a modified sample preparation technique that allows morphological examination of particles prior to polishing for quantitative analysis.
The part of the WDC06A drilled during the 2009/10 field season represents a time interval of abundant regional volcanism. This activity is reflected in the large number of visible dust bands and cloudy layers that were observed in the core (A. Orsi, pers. comm., 2010). Although no chemical analyses have been made, a distinct “visible brown layer” at a depth of 1586.363 m. (8.279 KyBP Neumann age) is very likely to be from a major eruption of the West Antarctic volcano Mt. Takahe. This eruption is dated at 8.2±5.4 using 40Ar/39Ar geochronology (Wilch et al., 1999) and is observed at a depth of between 503.58 and 503.87 m in the Siple Dome A core(SMDA) corresponding to ages between 8.167 and 8.181 Ky before 1950. A layer almost certainly corresponding to this one was identified and analyzed in the Byrd ice core at a depth of 788 m (Palais et al., 1988). A visible double layer at 1741.246 m (9.57 KyBP Neumann age) may correspond to a very distinct tephra layer in the SDMA core at a depth of around 550 m (corresponding to an age of around 9.7 Ky before 1950). This layer is derived from West Antarctic stratovolcano, Mt. Berlin. In the next segment of WDC06A ice core, between 2251 and 2557 m depth (15.2 to 20.6 KyBP Neumann age), numerous dust bands and cloudy layers are reported in the ice. This corresponds to the age of ice in the Byrd Core that contained many volcanic layers (Gow and Williamson, 1971), and also an interval in the SDMA where numerous distinct tephra layers associated with highly explosive eruptions of Mt. Berlin. Detailed quantitative chemical analyses will be required to make one-to-one correlations between tephra layers in the WDC06A and SDMA cores, but once made, will allow one additional tool to tie the records in these two cores together.
References
-
Alley, R.B., Meese, D.A., Shuman, C.A., Gow, A.J., Taylor, K.C., Grootes, P.M., White, J.W.C., Ram, M., Waddington, E.D., Mayewski, P.A., and Zielinski, G.A., 1993, Abrupt increase in Greenland snow accumulation at the end of the Younger Dryas event: Nature, v. 362, p. 527-529.
-
Basile, I., Petit, J.R., Touron, S., Grousset, F.E., and Barkov, N., 2001, Volcanic layers in Antarctic (Vostok) ice cores: Source identification and atmospheric implications: Journal of Geophysical Research-Atmospheres, v. 106, p. 31915-31931.
-
Bender, M., Sowers, T., and Brook, E., 1997, Gases in ice cores, in Keeling, C.D., ed., National Academy of Sciences colloquium on Carbon dioxide and climate change: Papers from a National Academy of Sciences colloquium; Carbon dioxide and climate change: Washington, DC, National Academy of Sciences.
-
Brook, E.J., White, J.W.C., Schilla, A.S.M., Bender, M.L., Barnett, B., Severinghaus, J.P., Taylor, K.C., Alley, R.B., and Steig, E.J., 2005, Timing of millennial-scale climate change at Siple Dome, West Antarctica, during the last glacial period: Quaternary Science Reviews, v. 24, p. 1333-1343.
Crowley, T., 2000, Causes of climate change over the past 1000 years: Science v. 289, p. 270-277.
-
Curzio, P., Folco, L., Laurenzi, M.A., Mellini, M., and Zeoli, A., 2008, A tephra chronostratigraphic framework for the Frontier Mountain blue-ice field (northern Victoria Land, Antarctica): Quaternary Science Reviews, v. 27, p. 602-620.
-
Dixon, D., Mayewski, P., Kaspari, S., Kreutz, K., Hamilton, G., Maasch, K., Sneed, S., and Handley, M., 2004, A 200-year sulfate record from 16 Antarctic ice cores and associations with Southern Ocean sea ice extent: Annals of Glaciology v. 39, p. 545-556.
- Dunbar, N.W., Kurbatov, A., Zielinski, G., McIntosh, W.C., Price, P.B., and Bay, R.C., 2003, Volcanic ash records in the Siple Dome Ice Core: West Antarctic Ice Shelf 2003 Research Meeting.
- Dunbar, N.W., Kurbatov, A., Zielinski, G., McIntosh, W.C., Price, P.B., and Bay, R.C., 2004, Volcanic ash records in the Siple Dome Ice Core: Siple Dome Principal Investigator Meeting, Reno, NV, Feb. 24, 2003. Download Powerpoint
- Dunbar, N.W., McIntosh, W.C., V., K.A., and Wilch, T.I., 2007, Integrated tephrochronology of the West Antarctic region; implications for a potential tephra record in the West Antarctic ice sheet (WAIS) Divide ice core, in Cooper, A., and Raymond, C., eds., Tenth international symposium on Antarctic earth sciences; Antarctica; a keystone in a changing world, U. S. Geological Survey. Extended abstract
- DUNBAR, N., G. ZIELINSKI, and D. VOISINS, 2003, Tephra layers in the Siple and Taylor Dome Ice Cores, Antarctica: Correlations and Sources. Journal of Geophysical Research,108, 2374-2385.
-
Gow, A.J., and Williamson, T., 1971, Volcanic ash in the Antarctic ice sheet and its possible climatic implications: Earth and Planetary Science Letters, v. 13, p. 210-218.
-
HAWLEY, R. L., E. D. WADDINGTON, D. L. MORSE, N. W. DUNBAR, and G. A. ZIELINSKI, 2002, Dating firn cores by vertical strain measurements: Journal of Glaciology, V. 48, p410-406
- Kurbatov, A.V., Zielinski, G.A., Dunbar, N.W., Mayewski, P.A., Meyerson, E.A., Sneed, S.B., and Taylor, K.C., 2006, A 12,000 year record of explosive volcanism in the Siple Dome Ice Core, West Antarctica: Journal of Geophysical Research-Atmospheres, v. 111, p. D12307. View abstract
-
Lemieux-Dudon, B., Blayo, E., Petit, J.R., Waelbroeck, C., Svensson, A., Ritz, C., Barnola, J.M., Narcisi, B.M., and Parrenin, F., 2010, Consistent dating for Antarctic and Greenland ice cores: Quaternary Science Reviews, v. 29, p. 8-20.
-
Lowe, D.J., 2008, Globalization of tephrochronology: new views from Australasia: Progress in Physical Geography, v. 32, p. 311-335.
-
McIntosh, W.C. and Wilch, T.I., 1995b. Late Pleistocene (223-125 ka) Plinian eruptions in Marie Byrd Land: Potential time horizons in West Antarctic ice cores. VII International Symposium of Antarctic Earth Sciences, Siena, Italy. P. 261.
-
Narcisi, B., Petit, J.R., B., D., Basile-Doelsch, I., and V., M., 2005, Characteristics and sources of tephra layers in the EPICA-Dome C ice record (East Antarctica); implications for past atmospheric circulation and ice core stratigraphic correlations: Earth and Planetary Science Letters, v. 239, p. 253-265.
-
Narcisi, B., Petit, J.R., and Delmonte, B., 2010, Extended East Antarctic ice-core tephrostratigraphy: Quaternary Science Reviews, v. 29, p. 21-27.
-
Narcisi, B., Petit, J.R., and Tiepolo, M., 2006, A volcanic marker (92 ka) for dating deep east Antarctic ice cores: Quaternary Science Reviews, v. 25, p. 2682-2687.
-
Nereson, N.A., Waddington, E.D., Raymond, C.F., and Jacobson, H.P., 1996, Predicted age-depth scales for Siple Dome and inland WAIS ice cores in west Antarctica: Geophysical Research Letters, v. 23, p. 3163-3166.
-
Oppenheimer, C., 2003, Ice core and paleoclimatic evidence for the timing and nature of the great mid-13th century volcanic eruption: International Journal of Climate, v. 23, p. 417-426.
-
Palais, J.M., Kyle, P.R., McIntosh, W.C., and Seward, D., 1988, Magmatic and phreatomagmatic volcanic activity at Mt. Takahe, West Antarctica, based on tephra layers in the Byrd Ice Core and field observations at Mt. Takahe: Journal of Volcanology and Geothermal Research, v. 35, p. 295-317.
-
Perchiazzi, N., Folco, L., and Mellini, M., 1999, Volcanic ash bands in the Frontier Mountain and Lichen Hills blue-ice fields, northern Victoria Land: Antarctic Science, v. 11, p. 353-361.
-
Robock, A., 1981, A latitudinally dependent volcanic dust veil index, and its effect on climate simulations.: Journal of Volcanology & Geothermal Research, v. 11, p. 67-80.
-
Robock, A., and Free, M., 1995, Ice cores as an index of global volcanism from 1850 to the present. : Journal of Geophysical Research- Solid Earth, v. 100, p. 11549–11568.
-
Sato, M., Hansen, J., McCormick, M., and Pollack, J., 1993, Stratospheric aerosol optical depths, 1850-1990.: Journal of Geophysical Research- Solid Earth, v. 98, p. 22987-22994.
-
Steig, E., Brook, E., White, J., Sucher, C., Bender, M., Lehman, S., Morse, D., Waddington, E., and Clow, G., 1998a, Synchronous climate changes in Antarctica and the North Atlantic: Science, v. 282, p. 92-95.
-
Steig, E.J., Morse, D.L., Waddington, E.D., and Polissar, P.J., 1998b, Using the sunspot cycle to date ice cores: Geophysical Research Letters ;, v. 25, p. 163-166.
-
Steig, E.J., Morse, D.L., Waddington, E.D., Stuiver, M., Grootes, P.M., Mayewski, P.A., Twickler, M.S., and Whitlow, S.I., 2000, Wisconsinan and Holocene climate history from an ice core at Taylor Dome, western Ross Embayment, Antarctica: Geografiska Annaler, v. 82, p. 213-235.
-
Stothers, R.B., 2001, A chronology of annual mean effective radii of stratospheric aerosol from volcanic eruptions during the twentieth century as derived from ground-based spectral extinction measurements. : Journal of Geophysical Research- Solid Earth, v. 106, p. 32043–32049.
- Wilch, T.I., McIntosh W.C., and Dunbar, N.W., (1999) Late Quaternary volcanic activity in Marie Byrd Land: potential 40Ar/39Ar -dated time horizons in future West Antarctic ice and marine cores, Geol. Soc. Am. Bull., 111, 1563-1580. ABSTRACT
-
Wolff, E., Basile, I., Petit, J.R., and Schwander, J., 1999, Comparison of Holocene electrical records from Dome C and Vostok ; Antarctica: Annals of Glaciology v. 29, p. 89-93.
-
Yalcin, K., Wake, C., Kreutz, K., Germani, M., and Whitlow, S., 2006, Ice core evidence for a second volcanic eruption around 1809 in the Northern Hemisphere: Geophysical Research Letters v. 33, p. L14706.
-
Zielinski, G.A., 1995, Stratospheric loading and optical depth estimates of explosive volcanism over the last 2100 years derived from the Greenland Ice Sheet Project 2 ice core: Journal of Geophysical Research, v. 100, p. 20937-20955.
-
Zielinski, G.A., Mayewski, P.A., Meeker, L.D., Gronvold, K., Germani, M.S., Whitlow, S.I., Twickler, M.S., and Taylor, K.C., 1997, Volcanic aerosol records and tephrochronology of the Summit, Greenland, ice cores: Greenland Summit ice cores; Greenland Ice Sheet Project 2, v. 102, p. 26,625-26,640.
- Zielinski, G.A., Mayewski, P.A., Meeker, L.D., Whitlow, S., and Twickler, M.S., 1996, A 110,000-yr record of explosive volcanism from the GISP2 (Greenland) ice core: Quaternary Research, v. 45, p. 109-118. ZIELINSKI., N. DUNBAR, A. KURBATOV, AND D. VOISINS, 2001, Holocene volcanic records in the Siple Dome Ice Core: EOS, Transactions of the American Geophysical Union, v. 82, p. F1408.
- Zielinksi, G., and N. Dunbar, 1998, Volcanic Record from the Siple and Taylor Domes, Antarctica: WAISCORES Meeting, Sept. 10, 1998.
Any opinions, findings and conclusions or recomendations expressed in this material are those of the author(s) and do not necessarily reflect the views of the National Science Foundation (NSF)
Return to HOME