
What is an Electron Microprobe and what can it do?
What is an electron microprobe?
An electron microprobe is a high powered microscope that uses electrons, rather than light, to examine a sample. Because electron are charged particles, they can be accelerated towards, and focused onto, a sample surface.
What can an electron microprobe do?
Electron microprobes can be used to produce morphological (roughness and shape) or chemical images of a sample surface. The microprobe can also be used to qualitatively or quantitatively determine the chemical composition of a very small spot (1 micron) on a sample surface. The electron microprobe can be used to chemically characterize samples so small that they can be analysed by virtually no other analytical technique, and also allows spatial resolution of chemical variability on a sample surface.
The capabilities of an electron microprobe are fundamental to many types of research, including many aspects of geology, such ore deposition, environmental geochemistry, hydrology, petroleum research, igneous and metamorphic geochemistry, soil science, and sedimentology, as well as various aspects of biology and metallurgy.
Click on the text caption or the following pictures for an enlarged view and explanation
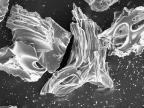
Secondary electron images
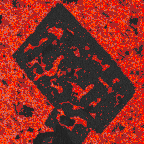
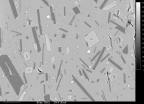

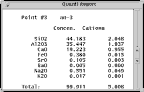
How does an electron microprobe work?
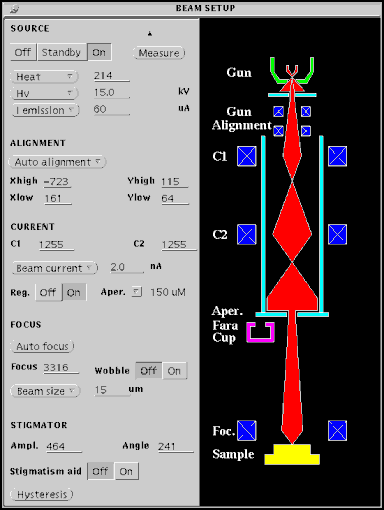
Electrons are produced by heating a tungsten filament to high temperatures (in the gun) and those electrons are accelerated away from the filament and gun towards the sample surface. Because the electrons are charged particles, they can be directed and focused using electronstatic lenses (gun alignment, C1, C2, foc, see left).
The electron beam is directed onto the sample surface, and can produce secondary electrons, backscattered electrons, cathodoluminescence, or X-rays that can be analysed to determine the sample's composition.
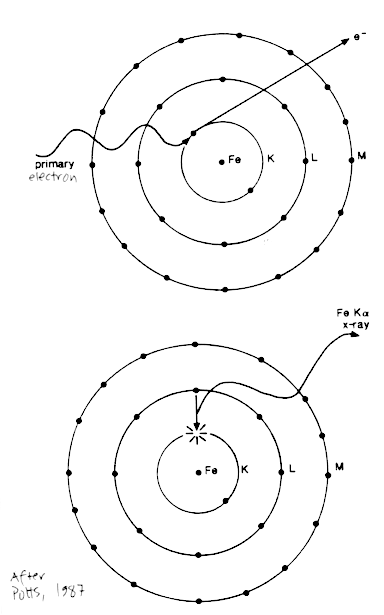
These include:
- Secondary electrons
- Backscattered electron
- Auger electrons
- Cathodoluminescnce
- Continuous X-rays
Interaction between the electron beam and the sample also produces characteristic X-rays, that have a wavelength and energy proportional to the atom from which they are produced. An electron from the primary beam causes an electron from the target atom to be ejected from its orbital shell. In response to the empty energy shell, another electron will cascade down to fill the lower energy position, in the process releasing energy in the form of a characteristic X-ray.
These characteristic X-rays can be detected by spectrometers to determined something about the sample composition.
The Cameca SX-100 is equipped with 3 wavelength-dispersive spectrometers, which are used to separate and detect characteristic X-rays generated from the sample. X-rays are diffracted by a curved crystals of known interlayer spacing (D) (shown in blue on figure), and then detected by a gas-flow-proportional counter.
Our spectrometers contain three types of crystals:
- TAP (best suited for light elements, between F and P)
- PET (best suited for intermediate Z elements, between Si and Fe)
- LIF (best suited for heavier elements, between K and As)
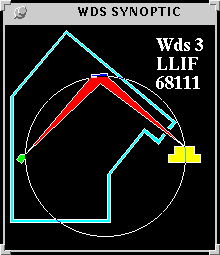