
What is X-ray Diffraction?
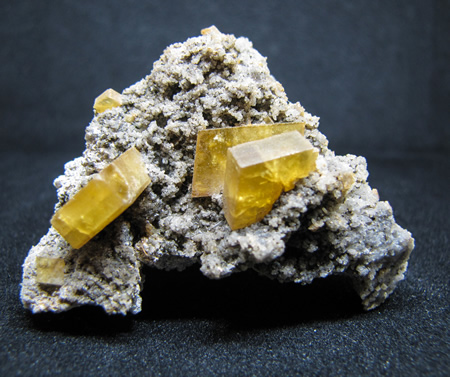
Photo by Kelsey McNamara
Barite, Day Tunnel, Pelican Mine, Hermosa District, Sierra Co., NM. Many geological samples do not have well-formed crystals like these.
Mineral identification is one of the most fundamental components of the geosciences. The types of minerals found in a rock or in different geologic settings can help geoscientists determine the geologic history of a region, the potential for developing economic resources, or the possibility of geologic hazards. Despite the numerous physical properties of minerals (color, luster, streak, crystal form, and cleavage), some samples are impossible to classify with the standard identification tools, such as the streak plate, scratch test, hand lens, and microscope. Every once in a while, someone will deliver a “head-scratcher” for mineral identification that we can’t figure out. These mystery minerals are typically poorly formed crystals or amorphous (lacking crystal structure), too small to see even with a hand lens or high-powered microscope, or a combination of the two. Luckily, there is yet another method for mineral identification—x-ray diffraction! In this article, we describe the x-ray diffraction (XRD) method and the XRD Laboratory at the New Mexico Bureau of Geology and Mineral Resources. But first, it might be a good idea to refresh on some basics back from high school physical science—x-rays and the electromagnetic spectrum.
X-rays and the electromagnetic spectrum
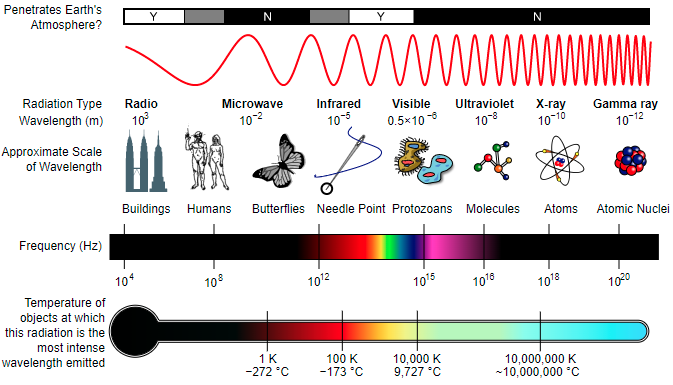
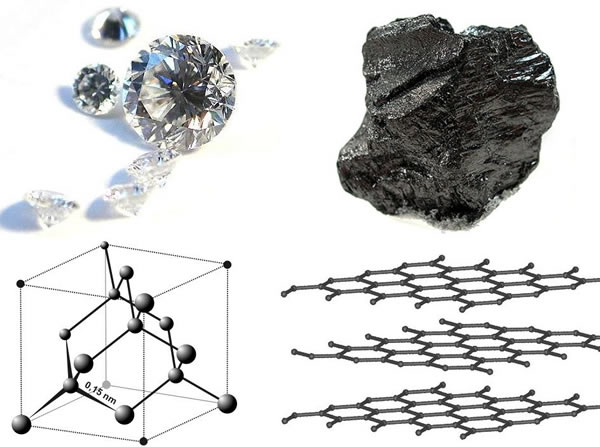
X-rays are a type of electromagnetic radiation (wave energy transmitted by variations in electric and magnetic fields) classified in the electromagnetic spectrum according to the energy level of individual photons, also expressed as wavelength. Also known as Röntgen radiation, they possess a wavelength range of ~0.1 to 10 nanometers (frequency of 3×1016 Hz to 3×1019 Hz). X-rays were produced and detected by a German scientist named Wilhelm Röntgen on November 8, 1895. He named it x-radiation, using the mathematical term “x” for something unknown.
To better clarify electromagnetic radiation, let’s compare visible light to x-rays. Both are forms of electromagnetic waves. However, our eyes are sensitive to the wavelength range of visible light, but not to shorter wavelength x-rays or longer wavelength electromagnetism (radio waves, for example). X-rays are most familiar to the public in their use in the medical industry, producing images of structures within the human body. They are absorbed in different amounts dependent on the density of the material they pass through. The soft tissue of your body is composed of smaller atoms, compared to the larger calcium atoms making up bones. X-rays largely pass through the small atoms of the soft tissue and are absorbed by the larger atoms of bones.
Since discovery, x-rays have been pivotal in the scientific advancement of medicine (radiography), physics, astronomy, and crystallography. X-rays are particularly useful in the study of crystallography—the branch of science focused on the physical structures of crystals—because their wavelengths closely match the distances between atomic planes (the layer of atoms) in crystalline substances.
Unlike the x-rays used in medicine, which are similar to white light (composed of a wide range of wavelengths), mineralogists use a special type of x-ray called monochromatic (single wavelength). To create monochromatic x-rays, a target metal is used to produce characteristic x-rays of the metal and metal filters are employed to screen out surrounding wavelengths. This target metal is most commonly copper, but iron, molybdenum, and cobalt are also used. The type of metal filter used depends on the type of monochromatic x-ray; for the element copper we use a nickel filter
Crystallography and x-ray diffraction (XRD)
Crystallography is the study of the arrangement of atoms in a crystalline solid in three-dimensional space. This arrangement is called a lattice and can be represented as dots uniformly placed in three-dimensional space (a two-dimensional lattice is a series of dots on a piece of paper). The planes represented by the dots (atoms) have different spacing between them depending on orientation. The number of atoms on any plane also changes with how they are oriented. The “regularity” of the spacing and ordering of the atoms determine how diffraction works in the lattice—producing large or small signals at different orientations.
It should be noted that a crystalline structure is not unique to just minerals; they form in a variety of materials such as alloys, semiconductors, salts, pharmaceuticals, and organic to inorganic molecules. X-ray diffraction has been paramount in many scientific fields to understand atomic structure and atomic-scale differences, as well as to provide data on physical and chemical properties in crystalline materials.
There are two types of XRD techniques, single crystal and powder. The difference between these methods is scale. As the name suggests, a single-crystal analysis is focused on exact atomic positions of a single well-ordered crystal, while powder XRD characterizes a sample of bulk material. We analyze powder samples at the New Mexico Bureau of Geology and Mineral Resources XRD Lab.
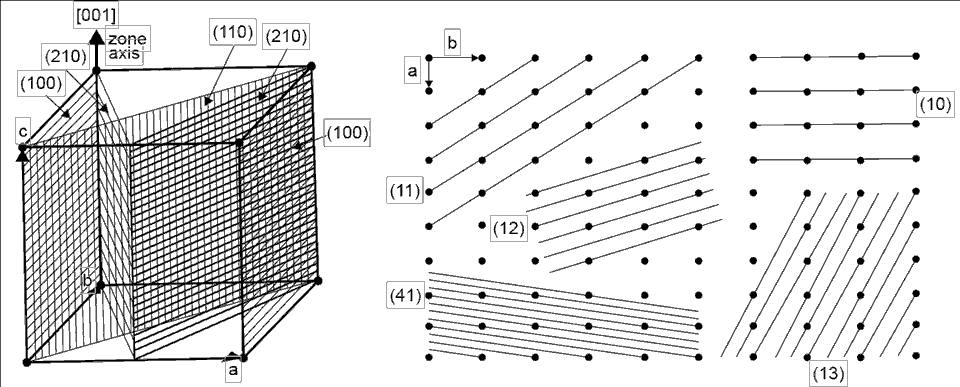
modified from: Cullity, B.E., 1978, Elements of X-Ray Diffraction, Second Edition, Addison-Wesley
Publishing Co., Inc., Reading, Massachusetts
Examples of lattice planes. (left) Planes in a cubic structure where atoms are at
each of the corners. Imagine more of these cubes stacked in all directions around
the example. (right) A two-dimensional lattice showing how the planes can be
oriented. Each four dots represent the face of a cube lattice. Crystallographers
identify each plane by using a standard coordinate system based on direction and
distance called Miller Indices.

Kelsey McNamara
An example of five powdered samples loaded into
holders and ready to be scanned. Note the variable
colors of the powders, which suggest different
mineral assemblages present in each sample.How does XRD instrumentation work?
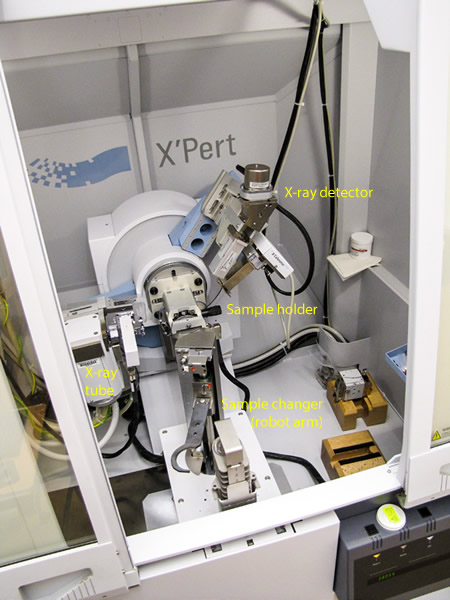
Kelsey McNamara
The interior of the Panalytical X’Pert Pro Diffractometer.
X-ray diffraction is performed by an x-ray diffractometer, which consists of several components including an x-ray tube, an x-ray detector, and a sample holder.
X-rays are produced in a cathode tube by heating a filament, which then emits electrons. These electrons are then accelerated toward a metal target, commonly composed of copper. When the electrons interact with the copper target, they lose energy in the form of monochromatic x-rays (a single wavelength). The produced x-rays are then collimated (made parallel to one another) and directed toward the sample.

modified from:"X-rays and ionizing radiation", Design World, 2015
Schematic diagram showing how x-rays are generated in an x-ray tube.
The x-ray tube and detector rotate around the sample while the intensity of the diffracted x-rays is recorded. The interaction of the x-rays with the geometry of crystallographic planes produces a characteristic XRD pattern called a diffractogram, which is used to identify minerals, measure crystallite size, determine thicknesses of atomic layers, recognize deformation of crystals, and other phenomena. To identify the mineral(s) present, a database of over 800,000 powder diffraction patterns is referenced. This method can be thought of as analogous to the same technique performed at a crime scene—fingerprints are collected and then compared to a fingerprint database to discern a match.
The New Mexico Bureau of Geology and Mineral Resources XRD Laboratory provides services to further research and education at New Mexico Tech, as well as serves agencies and industries of New Mexico by providing analyses that cannot be obtained through commercial sources. Each year a class is offered through the Earth and Environmental Science Department to teach students XRD analysis, including the subjects of x-ray generation and properties, crystallography, diffraction, and data interpretation. Many professors and graduate students across a number of disciplines conduct research in this lab. We estimate that 75% of the hands-on use of the equipment is by graduate and undergraduate students of New Mexico Tech.
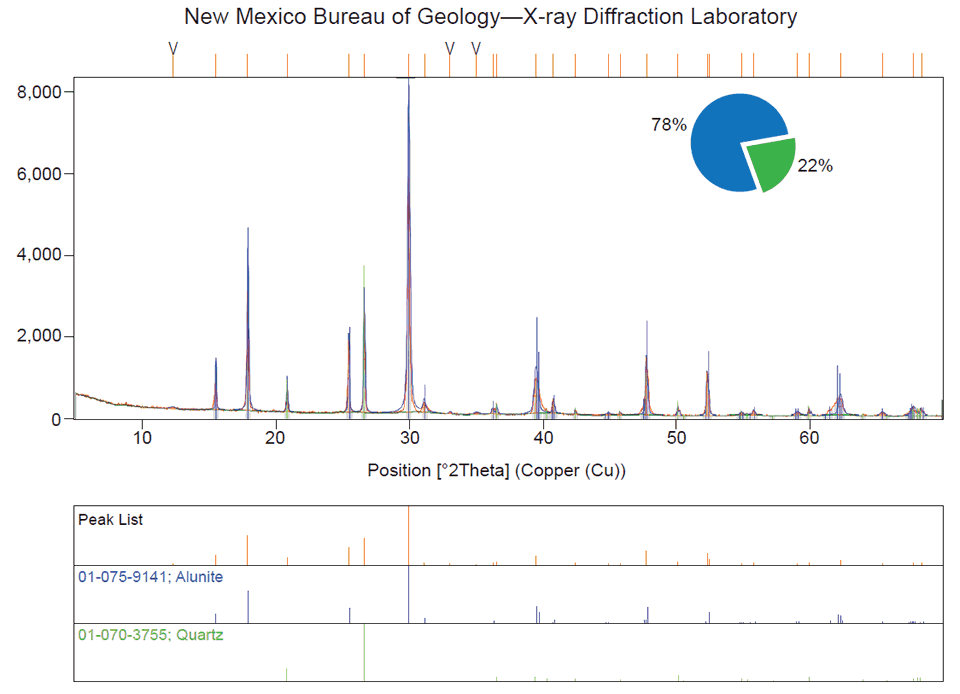