
Cumbres and Toltec Scenic Railroad
Introduction
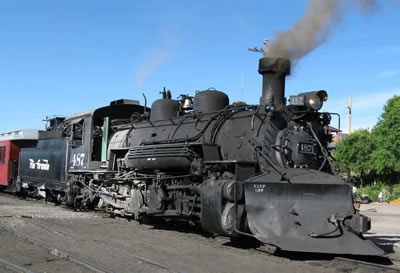
The 64-mile stretch of narrow gauge railroad track between Antonito, Colorado and Chama, New Mexico was originally built in 1880 as part of the Denver and Rio Grande Western Railroad. This rail line provided much-needed transportation and freight service between Denver and mining camps in Silverton during the late nineteenth and early twentieth centuries. When the Federal Government discontinued the use of the silver and gold standard to back American currency, the "Silver Panic" in 1893 caused the closure of many of the mines in the Silverton area. The railroad continued to operate with revenues from transportation of livestock, timber, and farm produce. The oil and gas industry in the Four Corners region also utilized the railroad. Demand for rail transportation in this region waned by the mid-twentieth century. Passenger service on the Denver and Rio Grande Western Railroad ended in 1951 and freight service ended in 1968. Railroad enthusiasts and legislative bodies in New Mexico and Colorado recognized the scenic splendor of the train route between Chama and Antonito. Through a joint effort, the Cumbres and Toltec Scenic Railroad, complete with coal-powered steam engines (Figure 1) carrying tourists in railcars on refurbished narrow gauge tracks, was created in 1970 to preserve this historic and picturesque section of railroad.
Regional Geologic Setting
The journey between Chama and Antonito traverses the San Juan Basin, the Tusas Mountains, and the San Luis Basin (Figure 2). The San Juan Basin on the Colorado Plateau is an important oil-producing basin that formed during compressional deformation 75 to 55 million years ago. This compressional event, known as the Laramide mountain building event, affected the entire western United States, creating large basins and mountain ranges. The Tusas Mountains also formed during Laramide deformation and are part of the southern Rocky Mountains. Ancient rocks that are 1.4 to 1.7 billion years old were brought toward the surface along faults and were exposed by erosion between 75 and 55 million years ago.
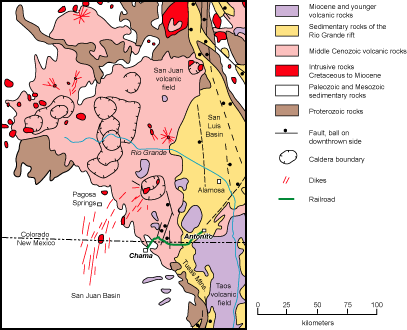
The Tusas Mountain highland and the San Juan Basin were then partially covered by lava flows and ash-flow tuffs derived from cataclysmic eruptions of 38-to-19 million-year-old volcanoes in the San Juan Mountains to the north (Figure 2). Andesitic stratovolcanoes dominated the landscape during the early history of the volcanic field. Later, several violent caldera-forming eruptions in the San Juan Mountains blanketed large portions of southwestern Colorado and north-central New Mexico with rhyolitic ash-flow tuff (Figure 2). Younger volcanic debris eroded from the volcanoes later lapped onto the northeast margin of the Tusas Mountains.
As the volcanoes in the San Juan Mountains eroded, this part of New Mexico and Colorado was stretched by east-west extension, causing eruption of basalts and the formation of deep sediment-filled basins (Figure 2). This extensional feature, known as the Rio Grande rift, started forming in this area about 25 million years ago. The youngest rift-related basaltic volcanism in this region (1 to 5 million years old, Appelt, 1998) is in the Taos Plateau volcanic field in the vicinity of Antonito.
Geologic History
The oldest rocks visible along the railroad route are exposed in Toltec Gorge in the core of the Tusas Mountains. Manley and Wobus (1985) recognized two distinct rock types in the gorge. The older unit is an intermediate composition intrusive rock known as granodiorite that was emplaced deep in the crust about 1.7 billion years ago. While the rock was still hot, compressional mountain building associated with the southward growth of the North American continent deformed the 1.7 billion-year-old intrusion, causing platy mica minerals in the rock to align and form a pronounced gneissic fabric in the rock. During this process, the igneous rock became a metamorphic rock called granodiorite gneiss. The mountains that formed as a result of this deformational event were aligned northeast-southwest. Later, a second plutonic body with a more granitic composition (technically called a quartz monzonite) intruded the older granodiorite gneiss about 1.4 billion years ago. The younger intrusion does not have a gneissic fabric, which suggests that the crust was not under compression at the time of emplacement.
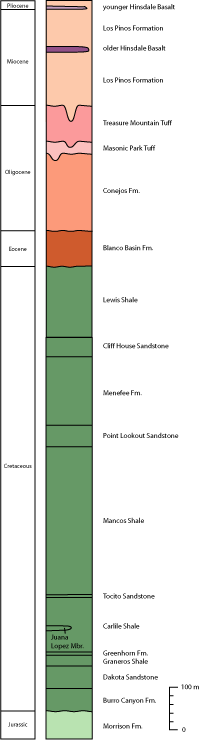
The geologic history of the area during the time interval between 1.4 billion years ago and about 150 million years ago is not preserved or is not exposed in the immediate vicinity of the railroad. Muehlberger (1967) proposed that the Tusas Mountains were a highland during late Paleozoic Ancestral Rocky Mountain deformation starting about 310 million years ago, based on bed thickness changes and fossils preserved in Pennsylvanian sedimentary rocks exposed along the western mountain front south of Chama. The late Paleozoic uplift was eroded and had subdued topography by late Triassic time about 225 million years ago because Chinle Formation laps onto Proterozoic rocks in the Tusas Mountains south of Chama (Muehlberger, 1967).
The oldest sedimentary rocks that can be viewed from the train are tan sandstones and green-to-red siltstone of the Jurassic Morrison Formation. These outcrops are located just north of the village of Chama where the sandstone forms prominent cliffs on the west side of the Rio Chama. Rivers flowing toward the northeast about 150 million years ago deposited the Morrison Formation.
The mesas around the village of Chama are composed of Cretaceous coastal plain, shoreline and marine units that were deposited along the western margin of the Western Interior Seaway approximately 125 to 70 million years ago (Figure 3). Approximately 25 million years of Earth's history is missing across the contact between the Late Jurassic Morrison Formation and the Early Cretaceous Burro Canyon Formation. The 100-to-125-million-year-old Burro Canyon Formation consists of crossbedded sandstone, quartz and chert pebble conglomerate, and pale-green to pale-red mudstones (Owen et al., 2005). The unit was deposited by braided streams flowing across a coastal plain towards the northeast to north, toward the Western Interior Seaway.
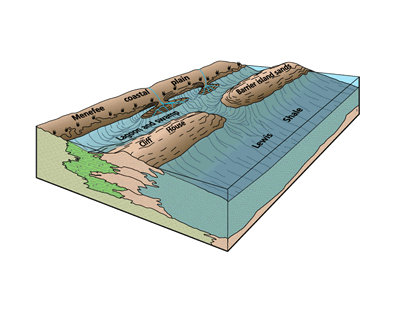
The Dakota Sandstone is composed of interbedded tan-to-yellow brown-weathering sandstone and dark gray carbonaceous shale and siltstone. The sandstones are locally cross-bedded, but, in general, the sandstones were intensely burrowed by marine organisms living in the shallow water along the shores of the Western Interior Seaway. Burrows are structures in sedimentary rocks formed by organisms digging or moving through sediment when the sand or mud was soft; organisms burrow through sediments seeking shelter, protection, or food. The Dakota Sandstone records the alternating rise (shale) and fall (sandstones) of sea level as the shoreline moved back and forth across the area about 98 to 100 million years ago. Gradual, long-term rise in sea level deposited rocks of Mancos Shale, a mud deposited on a shallow ocean floor about 98 to 80 million years ago. The shoreline then retreated, allowing deposition of the Point Lookout Sandstone along the shoreline and fluvial Menefee Formation in coal swamps along the edge of the seaway (Figure 4). Sea level rose once again, forming the sandy barrier island deposits of the Cliff House Sandstone and the muddy open marine deposits of the Lewis Shale. The sea retreated from New Mexico about 70 million years ago.
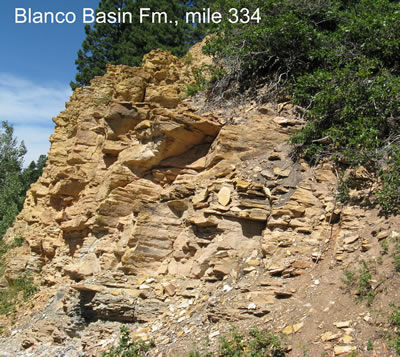
During the waning stages of Western Interior Seaway deposition, plate tectonic forces began to shape the high-elevation landscape that we see today (see below). The San Juan Basin was depressed and the Tusas Mountains rose during Laramide deformation starting about 75 million years ago. The reddish-to-tan sandy mudstone, pebbly sandstone, and conglomerate of the Blanco Basin Formation (Figure 5) was deposited in a localized depression called the San Juan sag that developed north and east of the village of Chama during Eocene time (Brister, 1990). Sedimentary detritus deposited in the Blanco Basin Formation was derived from eroding Laramide highlands located to the north and east of Chama.
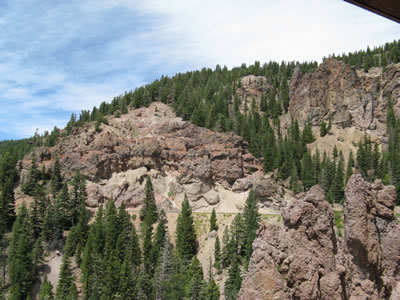
A few volcanoes erupted during Laramide time in the northwestern San Juan Mountains. Volcanic activity became quite intense in this area starting around 38 million years ago, peaking with the eruption of the voluminous Fish Canyon Tuff from the La Garita caldera 28 million years ago. Early andesitic stratovolcanoes across the volcanic field produced large volumes of breccia, lava, and volcaniclastic sediments (Figure 6 ; Colucci et al., 1991). Early silicic volcanism and associated caldera eruptions began 37 million years ago in the Sawatch Mountains northeast of the San Juan Mountains, and volcanism has generally migrated to the southwest toward the center of the San Juan Mountains (Lipman and McIntosh, 2008). The youngest tuff was erupted from the Lake City area about 23 million years ago (Lipman and McIntosh, 2008).
What triggered mountain building and volcanic activity in this area?
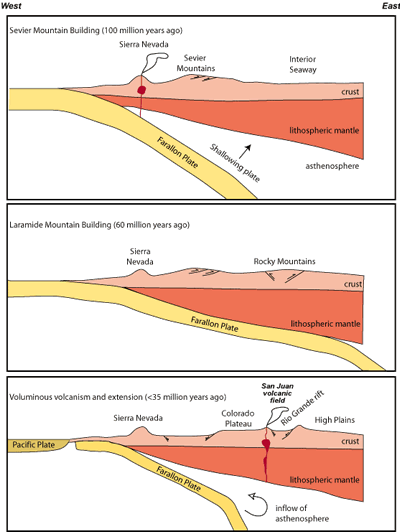
The mountains and the remnants of long-dormant volcanoes that we see today can be explained in terms of plate tectonics. According to one hypothesis, the geologic story of the Chama-Antonito area actually began nearly 100 million years ago and hundreds of miles to the west, when the Farallon Plate was subducted eastward under the western edge of the North American Plate (Figure 7). This plate, which was between the Pacific and the North American plates, has largely been consumed by subduction during the last 100 million years. Originally, the Farallon Plate was subducted beneath North America at a fairly steep angle, giving rise to the plutons preserved in the roots of the Sierra Nevada in California (top panel of Figure 7). Mountain building and magmatism migrated eastward and arrived in southwestern Colorado and northwestern New Mexico approximately 75 million years ago. This eastward migration is thought to have been caused by flattening of the subduction angle of the Farallon plate, initiated either by an increase in the rate of subduction or by subduction of a buoyant oceanic plateau. Between about 70 and 40 million years ago, the amount of magmatism dramatically decreased because the shallow subduction angle prevented dewatering of the slab and melting of the overlying mantle. Compression of the crust associated with low-angle subduction led to the formation of the Rocky Mountains during the Laramide mountain building event (middle panel of Figure 7).
Between 45 and 35 million years ago, subduction of the Farallon Plate slowed, and the angle of subduction started to increase. This area was still under mild compression as the early andesite stratovolcanoes in the San Juan volcanic field began to erupt. As the subducting plate continued to roll back or sink down into the mantle, hot asthenosphere came in contact with lithospheric mantle that had been enriched by fluids during the subduction process. As a consequence, the conditions were right for explosive and voluminous volcanism. Shortly thereafter, extension led to the formation of the Rio Grande rift (bottom panel of Figure 7).
Although Rio Grande rift extension mainly affected the eastern side of the Tusas Mountains and the western San Luis Basin in the vicinity of the railroad (Figure 2), numerous northwest-striking normal faults have been recognized in the Tusas Mountains between Chama and Antonito (Manley, 1982; Manley and Wobus, 1985; Manley et al., 1987). Ash flow tuffs from the San Juan Mountains were deposited across the area now occupied by the San Luis Basin; the tuffs have been down-dropped and tilted by down-to-the east normal faults. The tuffs have been buried by rift-fill sediments of the Los Pinos Formation, which were derived primarily from the eroding San Juan volcanic field. Rift-related basaltic lavas belonging to the Hinsdale Basalt erupted from 27 to 15 million year old volcanic centers along the east side of the Tusas Mountains and from the 5 million year old Los Mogotes volcano to the northwest of Antonito (Lipman and Mehnert, 1975). The Hinsdale Basalt is interbedded with the volcaniclastic Los Pinos Formation (Figure 3).
The combination of the eruption of large volumes of basalt in the Taos volcanic field near Antonito between 1 and 5 million years ago and uplift of the San Luis Hills northeast of Antonito by Rio Grande rift faulting blocked southward drainage of water from the San Luis Basin starting around 3 million years ago. As a result, a large lake called Lake Alamosa that was 30 miles wide in an E-W direction and 60 miles long in a N-S direction covered the floor of the basin north of the San Luis Hills (Machette et al., 2007). The lake was 200 feet deep in places. The lake overtopped the San Luis Hills and drained about 440,000 years ago (Machette et al., 2007).
The San Juan Mountains of Colorado were affected by two episodes of Ice Age glaciation. The first big glaciation, called Bull Lake by geologists, happened 130,000 to 95,000 years ago (Leonard, 1984). The more recent glaciation, known as the Pinedale, started 21,000 to 23,500 years ago (Leonard, 1984). The glaciers reached their maximum extent about 18,000 years ago and the big San Juan Mountain glaciers were melted and gone by 15,000 years ago (Guido et al., 2007). During times of maximum glaciation, all but the highest peaks along the Continental Divide in the headwaters of the Rio Grande, Animas River, Rio Chama, and the Lake Fork of the Gunnison River were covered with ice fields (Atwood and Mather, 1932; Carrara et al., 1984; Leonard, 1984). Glaciers also filled the valleys on both sides of the Continental Divide.
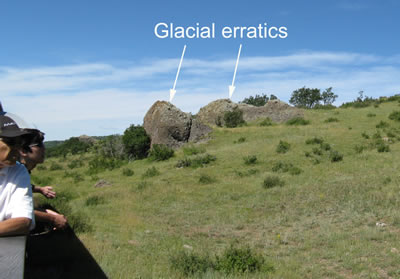
Drainages between Chama and Cumbres Pass along the route of the railroad contained valley glaciers. Ice is very efficient at eroding and carrying rock from the bottom and sides of a valley. Furthermore, rock debris tumbles onto the top of the glaciers from the sides of the valley. Consequently, glaciers contain a lot of rock debris. When the ice melts, the rock debris, which can range in size from house-sized blocks to flour-sized particles, drops out of the ice. The debris often forms ridges called moraines at the end of the glacier (terminal or recessional moraines) or along the valley margins of the glacier (lateral moraines). Large blocks of rock carried long distances from their point of origin by glaciers are called erratics (Figure 8).
References
- Appelt, R. M. (1998) 40Ar/39Ar geochronology and volcanic evolution of the Taos Plateau volcanic field, northern New Mexico and southern Colorado: Unpublished M.S. thesis, New Mexico Institute of Mining and Technology, 149 pp.
- Atwood, W.W., and Mather, K.F. (1932) Physiography and Quaternary geology of the San Juan Mountains, Colorado: U.S. Geological Survey Professional Paper 166, 176 pp.
- Brister, B.S. (1990) Tertiary sedimentation and tectonics: San Juan sag-San Luis Basin region: Ph.D. dissertation, New Mexico Institute of Mining and Technology, 267 pp.
- Burroughs, R. L. and Butler, A. P. (1971) Third Day Rail Log: Antonito, Colorado, to Chama, New Mexico, in James, H. L., ed., Guidebook of the San Luis Basin, Colorado: New Mexico Geological Society, p. 41-67.
- Carrara, P. E., W. N. Mode, M. Rubin, and S. W. Robinson (1984) Deglaciation and postglacial timberline in the San Juan Mountains, Colorado: Quaternary Research, v. 2 1, no. 1, p. 42-55.
- Colucci, M.T., Dungan, M.A., Ferguson, K.M., Lipman, P.W., and Moorbath, S. (1991) Precaldera lavas of the southeast San Juan Volcanic field: Parent magmas and crustal interactions: Journal of Geophysical Research, v. 96, p. 13,413-13,434.
- Humphreys, E., Hessler, E., Dueker, K., Farmer, C., Erslev, E., and Atwater, T. (2003) How Laramide-age hydration of North American lithosphere by the Farallon slab controlled subsequent activity in the western United States: International Geology Review, v. 45, p. 575–595.
- Kelly, S., Barkmann, P, Benson, R., Lovekin, J., Dunn, L., 2021, Geologic road log: Cumbres and Toltec scenic railroad, New Mexico Bureau of Geology & Mineral Resources, 35 p. ISBN: 978-1-883905-50-7
- Leonard, E. M. (1984) Late Pleistocene equilibrium-line altitudes and modern snow accumulation patterns, San Juan Mountains, Colorado: U.S.A. Arctic and Alpine Research v. 16, p.65–76.
- Lipman, P.W. (2006) Geologic map of the central San Juan Cluster, southwestern Colorado. U.S. Geological Survey Map I-2799.
- Lipman, P. W. and McIntosh, W. C. (2008) Eruptive and noneruptive calderas, northeastern San Juan Mountains, Colorado: Where did the ignimbrites come from?: Geological Society of America Bulletin, v. 120, p. 771–795.
- Lipman, P.W., and Mehnert, H.H. (1975) Late Cenozoic basaltic volcanism and development of the Rio Grande depression in the southern Rocky Mountains: Geological Society of America Memoir 144, p. 119-154.
- Guido, Z.S., Ward, D.J., and Anderson, R.S. (2007) Pacing the post--Last Glacial Maximum demise of the Animas Valley glacier and the San Juan Mountain ice cap: Geology, v. 35, p. 739-742.
- Machette, M.N., Marchetti, D.W., and Thompson, R.A. (2007) Ancient Lake Alamosa and the Pliocene to Middle Pleistocene evolution of the Rio Grande: U.S. Geological Survey Open-file Report OF07-1193, Chapter G, p. 157-167.
- Manley, K. (1982) Geologic map of the Bighorn Peak quadrangle, Rio Arriba County, New Mexico and Conejos County Colorado: U.S. Geological Survey Map MF-1451, 1:24,000 scale.
- Manley, K., and Wobus, R.A. (1985) Reconnaissance geologic map of the toltec mesa quadrangle, Rio arriba County, New Mexico: U.S. Geological Survey Map MF-1624, 1:24,000 scale.
- Manley, K., Scott, G.R., and Wobus, R.A. (1987) Geologic map of the Aztec 1° x 2° quadrangle, northwestern New Mexico and southern Colorado: U.S. Geological Survey Map I-1730, 1:250,000 scale.
- Muehlberger, W.R. (1967) Geology of Chama quadrangle, New Mexico: New Mexico Bureau of Mining and Mineral Resources Bulletin 89, 114 pp.
- Owen, D.E., Forgas, A.M., Miller, S.A., Stelly, R.J., and, Owen, D.E., Jr., 2005, Surface and subsurface stratigraphy of the Burro Canyon Formation, Dakota Sandstone, and intertongued Mancos Shale of the Chama Basin: New Mexico Geological Society Guidebook 56, p. 218-226.