
Cimarron Canyon State Park
modified from McLemore, V.T., 1990, Cimarron Canyon State Park: New Mexico Geology, v. 12, no. 3, p. 66-71.
Introduction
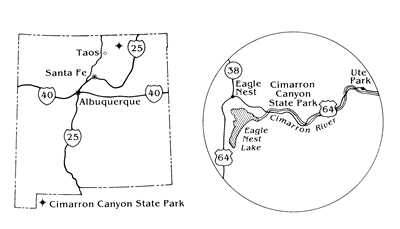
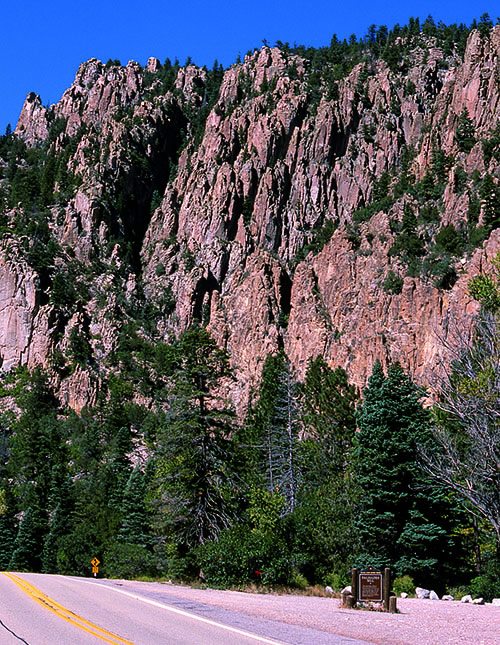
L. Greer Price
Cimarron is Spanish for wild and untamed and originally was used in New Mexico to refer to the wild bighorn sheep, and later to the wild horses and cattle that once roamed throughout the north-central mountains (Pearce, 1965). Today, the sparsely populated Cimarron country in western Colfax County (Fig. 1) can still be described as wild and untamed with its rugged, timbered mountains (the Cimarron Range), towering cliffs (Fig. 2), and the previously unpredictable Cimarron River. The Cimarron River has been tamed somewhat by the Eagle Nest Dam, which controls flooding in the canyon.
Cimarron Canyon State Park extends along Cimarron Canyon from Eagle Nest Lake to Ute Park and along US-64 (Fig. 1). It is part of Colin Neblett Wildlife Area, which consists of 33,116 acres of the central Cimarron Range of the southern Rocky Mountains. The wildlife area is bordered by the Philmont Boy Scout Ranch on the east and Eagle Nest Lake on the west. The elevation ranges from 7,400 ft along the river to 12,045 ft at Touch-Me-Not Mountain. The state park is managed jointly by the State Park and Recreation Division and the New Mexico Department of Game and Fish; the latter administers the wildlife area. Local wildlife includes deer, elk, bear, antelope, turkey, chipmunk, squirrel, beaver, coyote, red fox, porcupine, raccoon, bobcat, mountain lion, and a few bighorn sheep. Golden and bald eagles and other birds may be seen in the area.
Geology
Cimarron Canyon exposes nearly two billion years of complex geologic history. The canyon separates the older Proterozoic rocks to the south from younger Tertiary rocks to the north (Fig. 3). The oldest rocks in the area are Proterozoic metamorphic and igneous rocks.
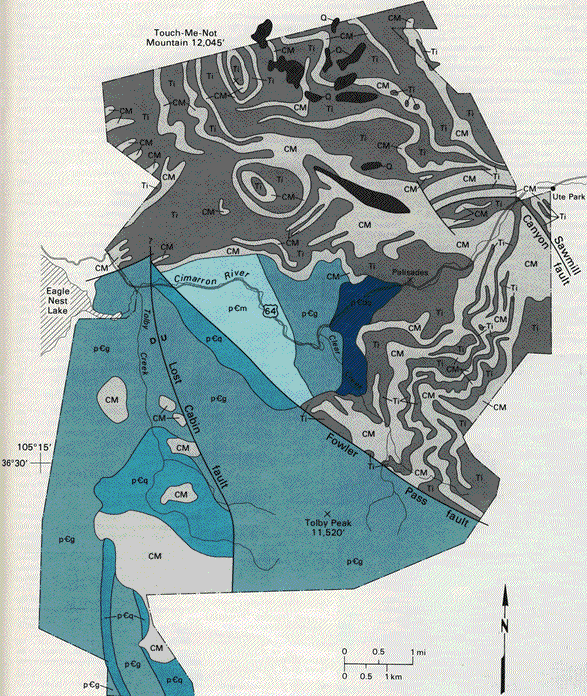
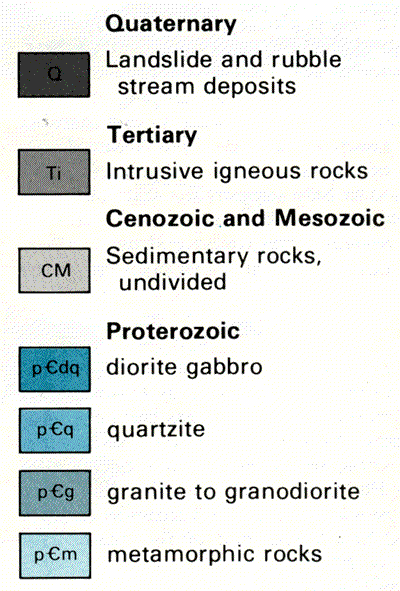
Two distinct terranes are separated by the Fowler Pass fault (Wobus, 1989). The rocks on the northeast side of the fault include felsic metamorphic rocks, phyllite, schist, and metasiltstone that have been intruded by Proterozoic stocks of gabbro to diorite to granodiorite and granite (pCdq, pCb, pCg, Fig. 2). Dark outcrops of the gabbro (pCdq, Fig. 2) crop out west of the Palisades (Wobus, 1989). The rocks on the southwest side of the fault include quartzite, amphibolite, and granite. These were once sedimentary or igneous rocks that were heated and squeezed under tremendous pressure deep within the earth's crust (metamorphosed) forming the lineation and banding characteristic of many of them. They consist predominantly of quartz, feldspar, hornblende, and minor amounts of biotite and epidote. The granite southwest of Fowler Pass fault has been dated as Middle Proterozoic, about 1.4 to 1.5 billion years old (Brookins and Leyenberger, 1981; Leyenberger, 1983). The metamorphic rocks are only slightly older, geologically speaking, between 1.6 and 1.9 billion years.
Only remnants of the thick sequence of sedimentary rocks (CM, Fig. 2) that were deposited from Pennsylvanian (320 million years ago) through early Tertiary (57 million years ago) are exposed in the wildlife area because Tertiary sills (Ti, Fig. 2) have intruded and disrupted the sequence. However, geologists can still speculate on the geologic history during the Pennsylvanian through early Tertiary Periods by studying more complete rock sequences elsewhere along the eastern slopes of the southern Rocky Mountains (Robinson et al., 1964; Smith and Ray, 1943).
Red and gray conglomerate, sandstone, shale, and gray limestone belonging to the Sangre de Cristo Formation (PennsylvanianPermian, about 320-245 million years ago) were deposited unconformably on top of the Proterozoic rocks. The younger rocks were deposited by streams and rivers. The red color comes from oxidation of magnetite grains that forms a thin coating of red hematite or limonite on the individual mineral grains within the rock.
The Chinle Formation (Triassic, about 245-208 million years ago) unconformably overlies the Sangre de Cristo Formation and consists of red, brown, tan, and green shale, siltstone, and finegrained sandstone. These rocks were also deposited by streams and rivers. The finer grain size of the sediments in the Chinle Formation compared to those in the Sangre de Cristo Formation suggests that the source of the younger sediments was farther away than the source of the Sangre de Cristo sediments.
The beginning of the Jurassic Period (about 208 million years ago) marks a time of change to a drier, more arid climate with the deposition of the Entrada Sandstone, a light-gray, massive sandstone. The crossbedding and rounded, frosted quartz grains are similar to those found in sand dunes typical of many modern deserts; this suggests to geologists that the sandstone was formed by similar dunes millions of years ago. The Jurassic Morrison Formation unconformably overlies the Entrada Sandstone and consists of red, gray, and brown shale, sandstone, siltstone, and thin gray limestone. This unit was deposited by streams and rivers.
During the Cretaceous Period (about 125 - 66 million years ago), shallow seas covered much of New Mexico. The Dakota Sandstone consists of red, gray, and brown sandstone with thin interbeds of shale that were deposited in a shallow marine beach setting. The black shales and gray limestones overlying the Dakota Sandstone belong, in ascending order, to the Graneros Shale, Greenhorn Limestone, Carlile Shale, Niobrara Formation, and Pierre Shale. The rocks were deposited as mud at the bottom of the sea so marine plant and animal fossils are sometimes found in them. Eventually the seas receded, leaving a continental coastal and alluvial plain (McLemore, 1990), but these rocks are not exposed in Cimarron Canyon.
After deposition of most of the sedimentary rocks, the Cimarron Range along with most of the Southern Rocky Mountains were uplifted during Laramide deformation, the period of mountain building and magmatic activity that occurred about 75-40 million years ago. Igneous sills and dikes (Ti, Fig. 2) were probably intruded into the sedimentary rocks at this time. Fowler Pass, Sawmill Canyon, and other faults were formed during this mountain uplift (Goodknight, 1976).
The Palisades in the eastern part of Cimarron Canyon State Park are one of the most spectacular geologic features in northern New Mexico. These cliffs were formed by a light-gray, fine-grained Tertiary sill (Ti, Fig. 2) that intruded the Cenozoic and Mesozoic sedimentary rocks (CM, Fig. 2) and Proterozoic metamorphic rocks (pCm, Fig. 2). This sill was dated by K/Ar methods as 34.7 million years old by Armstrong (1969); however, more recent studies indicate the sill is as young as 26 million years (Mutschler et al., 1987; Kish et al., 1990). 40Ar/39Ar ages on dikes and sills to the northeast of Cimarron Canyon State Park are 25 to 26 Ma (Miggins, 2002; Miggins et al., 2002).
Geologists sometimes have difficulty naming fine-grained or aphanitic igneous rocks because the minerals that compose the matrix are not easily identifiable. The Tertiary sill that forms the Palisades and Touch-MeNot Mountain consists of phenocrysts of plagioclase, biotite, hornblende, and quartz surrounded by a fine-grained, gray matrix. This texture is called porphyritic and the rock name includes the word porphyry. Based on mineralogy and chemical composition, the Palisades consist of biotite-diorite porphyry (Armstrong, 1969). Other geologists have called the rock type of the Palisades a monzonite porphyry (Lindgren et al., 1910), quartz monzonite porphyry (Smith and Ray, 1943), dacite porphyry (Robinson et al., 1964; Cannon, 1976), a granodiorite porphyry (Goodknight, 1973), or a transition from trachydacite to dacite (Kish et al., 1990). Although these terms describe the rock properly according to its composition (de la Roche et al., 1980), some terms are inconsistent with its texture. Therefore, the term porphyritic dacite seems the best description of these sills.
The porphyritic texture is produced by the magma crystallizing in stages at shallow depths. The first stage cooled slowly so that the phenocrysts were formed. The cooling then speeded up producing the fine-grained matrix. During this second stage, the melt partially attacked and remelted the phenocrysts, thereby rounding them (Robinson et al., 1964). The long columns and Palisade towers were shaped from joints formed during crystallization and cooling, followed by uneven weathering. Water seeped into the cooling joints and fractures, froze and expanded in winter to enlarge the opening, then thawed. This sequence occurred over and over again for thousands of years. Sometimes the fractures were enlarged so much that eventually rocks fell. Remnants of some rock falls are found in the Cimarron River and at the rest stop at the Palisades.
The Cimarron Range was uplifted slowly throughout the middle and late Tertiary. Streams formed at the crest of the mountains and water slowly began to erode and carry away sand and rock material from the crest. Even today, the rivers and streams are slowly eroding the mountains; boulders and smaller rocks from the higher ridges and peaks are found in the streams and accumulate in wide spots in the canyons, such as Gravel Pit Lakes in Cimarron Canyon.
The Cimarron River is the only water course that has cut through the Cimarron Range (Robinson et al., 1964) because it has had more water with which to erode and cut through the mountains. The other streams in the Cimarron Range drain small areas at the crest of the mountains whereas the Cimarron River drains the entire Moreno Valley (Fig. 1), a large lowland area separating the Cimarron Range and the Sangre de Cristo Mountains.
The Cimarron River was able to capture the waters from the Moreno Valley as the result of a set of special circumstances (Robinson et al., 1964). Ellis (1935) suggested that the Moreno Valley was occupied by a huge glacier during the Tertiary; however, Ray (1940) and Smith and Ray (1943) could find no evidence to support the presence of a glacier. The Moreno Valley formed as the Cimarron Range and Sangre de Cristo Mountains were uplifted. Streams from both mountain ranges flowed into the valley and then southward toward the eastern plains. Near the end of the Tertiary, the southern end of the valley was blocked by lava flows. Trapped streams became sluggish and began to fill the closed basin. Swamps and lakes developed and the valley slowly filled with sediment. Eventually, streams breached the Cimarron Range at a low spot along the eastern hills and the present course of the Cimarron River was set.
High on Touch-Me-Not Mountain and other mountains in Cimarron country, one can find areas of bare, unvegetated rock surfaces, typically called talus slopes (Q, Fig. 2). These talus deposits were formed after the mountains were uplifted. The same sequence of weathering caused by water action that contributed to formation of the Palisades eventually caused the rock to break into angular boulders. These boulders do not move far unless they are on a steep slope. There they will move slowly downhill forming a long talus slope of broken rubble (Robinson et al., 1964).
There are a few mineral prospects located in Cimarron Canyon State Park and Colin Neblett Wildlife Area, but the history of them is unknown. They were probably developed in the late 1800's as part of the mining boom at Elizabethtown. The largest, the Horseshoe mine along US-64, consists of a short adit or tunnel dug into the hillside along quartz veins in Proterozoic metamorphic rocks. Pyrite, probably containing traces of gold, and malachite are found in dump samples. Other small prospects are scattered throughout the area (Leyenberger, 1983; Goodknight, 1973), but none of them ever produced economic quantities of ore. The area is protected from mineral exploration and development and has not been evaluated for its mineral resources.
References
- Armstrong, R. L., 1969, K-Ar dating of laccolithic centers of the Colorado Plateau and vicinity: Geological Society of America, Bulletin, v. 80, pp. 2081-2086.
- Brookins, D. G., and Leyenberger, T. L., 1981, Rb-Sr isochron ages of two Precambrian igneous rock units, Colfax County, New Mexico: Isochron/West, no. 32, pp. 21-24.
- Cannon, R. P., 1976, Petrology and geochemistry of the Palisades sill, New Mexico: M.S. thesis, University of North Carolina; New Mexico Bureau of Mines and Mineral Resources, Open-file Report 81, 90 pp.
- de la Roche, H., Leterrier, J., Grandclaude, P., and Marchal, M., 1980, A classification of volcanic and plutonic rocks using R,R2-diagram and major element analyses-its relationship with current nomenclature: Chemical Geology, v. 29, pp. 183- 210.
- Ellis, R. W., 1935, Glaciation in New Mexico: University of New Mexico Bulletin 276, Geol. Sec, v. 5, pp. 1-31.
- Goodknight, C. S., 1973, Structure and stratigraphy of the central Cimarron Range, Colfax County, New Mexico: Unpublished M.S. thesis, University of New Mexico, Albuquerque, 84 pp.
- Goodknight, C. S., 1976, Cenozoic structural geology of the central Cimarron Range, New Mexico: New Mexico Geological Society, Guidebook to the 27th field conference, pp. 137-140.
- Kish, S. A., Ragland, P. C., and Cannon, R. P., 1990, Petrochemistry of the Cimarron pluton, northern New Mexico; in Bauer, P. W., Lucas, S. G., Mawer, C. K., and McIntosh, W. C. (eds.), Tectonic development of the southern Sangre de Cristo Mountains, New Mexico: New Mexico Geological Society, Guidebook 41, pp. 341-347.
- Leyenberger, T. L., 1983, Precambrian geology of Cimarron Canyon, Colfax County, New Mexico: Unpublished M.S. thesis, University of New Mexico, 93 pp.
- Lindgren, L., Graton, L. C., and Gordon, C. H., 1910, The ore deposits of New Mexico: U.S. Geological Survey, Professional Paper 68, 361 pp.
- McLemore, V. T., 1990, Sugarite State Park: New Mexico Geology, v. 12, no. 3, pp. 38-42.
- Miggins, D.P., 2002, Chronologic, geochemical, and isotopic framework of igneous rocks within the Raton Basin and adjacent Rio Grande rift, south-central Colorado and norhtern New mexico, M.S. thesis, University of Colorado, Boulder, Colorado,417 pp.
- Miggins, D.P., Thompson, R.A., Pillmore,C.L. Snee, L.W., and Stern,C.R., 2002, Extension and uplift of the northern Rio Grande Rift: Evidence from 40Ar/39Ar geochronology from the Sangre de Cristo Mountains, south-central Colorado and northern New Mexico; in Menzies, M.A., Klemperer, S.L., Ebinger, C.J., and Baker, J., (eds.), Volcanic rifted margins, Geological Society of America, Special Paper 362, p. 47-64.
- Mutschler, F. E., Larson, E. E., and Bruce, R. M., 1987, Laramide and younger magmatism in Colorado-new petrologic and tectonic variations on old themes; in Drexler, J. W., and Larson, E. E. (eds.), Cenozoic volcanism in the southern Rocky Mountains updated: A tribute to Rudy C. Epis-Part II, Colorado School of Mines Quarterly, v. 82, pp. 1-64.
- Pearce, T. M., 1965, New Mexico place names, a geographical dictionary: University of New Mexico Press, Albuquerque, 187 pp.
- Pettit, R. F., Jr., 1946, Mineral resources of Colfax County, New Mexico: New Mexico Bureau of Mines and Mineral Resources, Open-file Report 15, 50 pp.
- Ray, L. L., 1940, Glacial chronology of the southern Rocky Mountains: Geological Society of America, Bulletin, v. 51, pp. 1851-1917.
- Robinson, G. D., Wanek, A. A., Hays, W. H., and McCallum, M. E., 1964, Philmont country, the rocks and landscape of a famous New Mexico ranch: U.S. Geological Survey, Professional Paper 505, 152 pp.
- Smith, J. F., Jr., and Ray, L. L., 1943, Geology of the Cimarron Range, New Mexico: Geological Society of America, Bulletin, v. 54, pp. 891-924.
- Wobus, R. A., 1989, Proterozoic supracrustal rocks and plutons of the Cimarron Canyon area, north-central New Mexico; in Grambling, J. A., and Tewksbury, B. J. (eds.), Proterozoic geology of the southern Rocky Mountains: Geological Society of America, Special Paper 235, pp. 111-118.
- Young, J. V., 1984, The state parks of New Mexico: Albuquerque, University of New Mexico Press, 160 pp.