
Mt. Taylor Volcanic Field
Introduction and Geologic Setting
Mount Taylor volcano, a prominent landmark that can be seen on the skyline west of Albuquerque, is located about 15 miles northwest of the town of Grants, New Mexico. Mount Taylor Peak, at an elevation of 11,301 feet, stands approximately one mile above the Rio San Jose 12 miles to the south (Hunt, 1938). Mount Taylor volcano is part of a larger, northeast-trending volcanic field that includes Mesa Chivato, a broad plateau located northeast of the cone (Figure 1), and Grants Ridge (Keating and Valentine, 1998; WoldeGabriel et al., 1999), located southwest of the cone. Basalt that caps Mesa Chivato and other mesas surrounding Mount Taylor makes up about 80% of the volume of the volcanic field (Perry et al., 1990). The Mount Taylor volcanic field lies on the southern flank of the San Juan Basin on the Colorado Plateau and straddles the extensional transition zone between the Colorado Plateau and the Rio Grande rift (Figure 2). The Mount Taylor volcanic field is considered to be part of the Jemez Lineament, a zone of young (< 5 million years old, Figure 3) volcanism aligned along an ancient suture in the 1.7 to 1.6 billion year old Proterozoic basement (Mayo, 1958, Laughlin et al., 1976; Lipman and Moench, 1972; Perry et al., 1990).
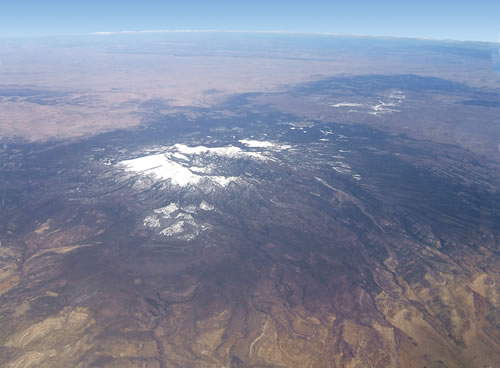
photo: © Kirt Kempter
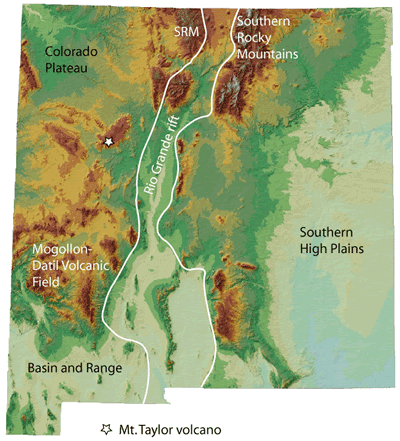
Geologic History
Pre-Volcanic History
Although Mount Taylor volcano is the feature that dominates the landscape near Grants, the earlier history of the region prior to the eruption of the volcanic rocks is well preserved in the bold cliffs below the volcanic rocks, particularly around the southern margin of the volcanic field. Sandstone and shale deposited along a shoreline, thick deposits of black shale deposited in an open ocean and tan sandstone with interbedded shale and coal deposited by a northeast-draining river system record the oscillation of the northwest-trending shoreline of the Cretaceous Western Interior Seaway back and forth across the area ~84 to 99 million years ago. The Cretaceous rocks exposed around Mount Taylor include the Dakota Sandstone, Mancos Shale, Gallup Sandstone, and Crevasse Canyon Formation (Lipman et al., 1979; Dillinger, 1990).
Following deposition of the Cretaceous rocks, the area was gently folded by Laramide compression starting about 80 million years ago (Cather, 2004). Mount Taylor is on a broad regional downwarp called the Acoma Sag (Crumpler, 1982). The McCarty syncline, a north-plunging broad symmetrical fold that extends from the east end of the Zuni Mountains north-northeast (N20°E) into the southern San Juan Basin, underlies the Mount Taylor volcanic field. At the south end of the field, the northward plunge of the fold dramatically increases and the west flank of the fold becomes a monocline, but at the north end of the field the syncline is no longer visible. (Hunt, 1938).
Volcanic history
Composite Cone
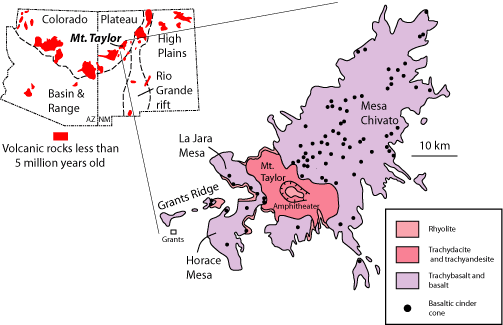
Mount Taylor is a composite rhyolitic to intermediate (trachytic) composition stratovolcano that was active >3.3 to 1.5 million years ago (Perry et al., 1990). Composite stratovolcanoes are usually made of lava flows interbedded with ash flow tuffs and pumice that form during explosive eruptions and volcaniclastic sediment (sediment related to erosion of the cone). Mount Taylor is a classic composite cone in nearly every respect (Figure 4) except that only 10% of the cone is made of tuff and pumice, a low volume compared to other volcanoes of this type (Perry et al., 1990). A 3.73 ± 0.09 million year old basanite flow sitting on Cretaceous sandstone at the north end of Horace Mesa is the oldest volcanic unit dated so far in the Mount Taylor field (Lipman and Moench, 1972). The oldest volcanic unit exposed in the bottom of the cone's amphitheater is a 3.3 million year old trachyte dome or flow. This trachyte dome is most likely related to trachytic volcanism to the northeast on Mesa Chivato (Crumpler, 1980, Lipman and Mehnert, 1979) and is not directly associated to the formation of the Mt. Taylor cone (Perry et al., 1990). After these initial eruptions, rhyolite (70 to 75% silica) lava erupted during the early history of the buildup of the volcanic cone, followed by trachydacite (63 to 70 % silica), and finally trachyandesite (58-63% silica) (Perry et al., 1990). In general, the composition of the lavas on the cone became more mafic through time. Most of the cone formed between 3 and 2.6 million years ago and trachydacite makes up more than 65 % of the cone (Baldridge et al., 1989). Basalt eruptions occurred through the lifetime of the volcano, although relatively little basalt erupted prior to the formation of the bulk of the cone. Most of the basalt in the Mount Taylor volcanic field erupted after the cone formed, erupting from vents around the volcano, with the basalt flows lapping onto the edge of the cone and spreading out over erosion surfaces (Hunt, 1938). Most of the basalt is trachybasalt (hawaiite), but flows ranging from basanite to trachyte in composition are also present (Lipman and Moench, 1972; Crumpler, 1977).
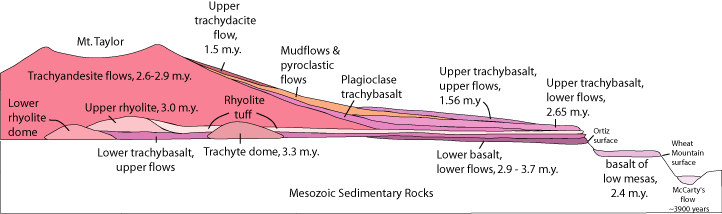
An east-facing amphitheater is a prominent feature in the central part of the volcano (Figures 1 and 3); Mount Taylor Peak is the highest point on the west rim of the amphitheater. Underlying Cretaceous sedimentary rocks and the oldest volcanic rocks in the cone are exposed in the lower elevations of the amphitheater. Crumpler (1982) suggested that the east side of Mount Taylor collapsed, leading to an lateral eruption like the 1980 eruption of Mount St. Helens in Washington state; however, no clear debris avalanche deposits indicative of a lateral eruption have been found on Mount Taylor (Perry et al., 1990). A volcaniclastic deposit containing boulders and cobbles of volcanic rock mantles the east side of the volcano (Lipman et al., 1979). Hunt (1938) and Perry et al. (1990) propose that the amphitheater is the result of erosional enlargement of a crater on Mount Taylor volcano.
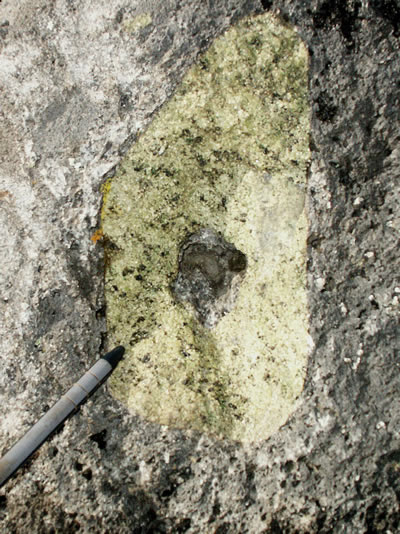
Perry et al. (1990) use spatial and temporal trends in the geochemistry of the lavas in conjunction with thin section and field observations to argue that several successive short-lived magma chambers, rather than one large chamber, fed Mount Taylor volcano. The trend of increased mafic content through time may be due to low rates of extension early in the history of the field, which may have allowed magma coming up from the mantle to pond in the crust and differentiate. Later in the history of the field, increased extension allowed more magma to pass through the crust with little time to differentiate. In fact, many of the later basalts contain abundant pieces of mantle rock (xenoliths; Figure 5) that were entrained in the magma, suggesting a relatively quick trip from the source in the mantle to eruption at the surface. These eruptions provide a wonderful opportunity to examine pieces of the Earth's mantle that formed at depths of 50 to 62 miles (80 to 100 km) below the surface (Pocerra et al., 2004).
Mesa Chivato
Mesa Chivato, located northeast of Mount Taylor, is the site of numerous fissure vents, collapse craters, maars, and trachyte domes (Aubele at al., 1976, Crumpler, 1980). Fissure vents are linear volcanic vents through which lava erupts, usually without any explosive activity. The vents are usually a few meters wide and may be many kilometers long. Maars are broad, low-relief volcanic craters formed by shallow explosive eruptions that are usually caused by the heating and boiling of groundwater when magma invades the groundwater table. Maars often subsequently fill with water to form a lake. Mesa Chivato is dotted by hundreds of basaltic vents that are aligned in both northeast and west-northwest directions (Figure 3). The orientation of the vents is thought to be controlled by underlying basement structures (Crumpler, 1982; Laughlin et al., 1982). In places, faults on the mesa follow northeast trending fissure vents and thus postdate the basalt. Elsewhere, fissure eruptions postdate faulting (Crumpler, 1980, 1982). The east side of the field is bounded by the Rio Puerco fault zone, including the Alamosa fault, San Ignacio monocline and Reservoir fault (Hunt, 1938), which form part of the eastern boundary of the Rio Grande rift.
A trachyte dome on Mesa Chivato yields a K-Ar age of 3.3 ± 0.2 million years (Lipman and Mehnert, 1979). This dome predates a 7.4 mile (12 km) wide zone of normal faulting that trends north-northeast across the mesa. As mentioned above, a trachyte flow or dome that is 3.3 ± 0.08 million year old (Perry et al., 1990) is exposed in the bottom of the Mount Taylor amphitheater. The domes on Mesa Chivato and Mt. Taylor formed at the same time and are compositionally similar, and thus may be related. Interestingly, the trachyte is not the oldest unit on Mesa Chivato. Several basalt flows and a basal rhyolite tuff lie below the dated flow, so the timing of the initiation of volcanism on Mesa Chivato is unconstrained.
Landscape Evolution
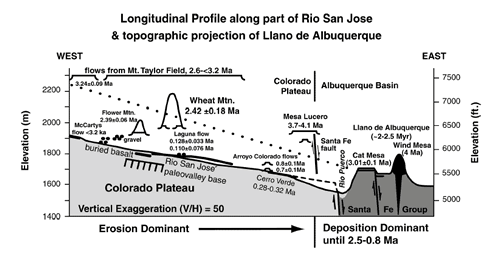
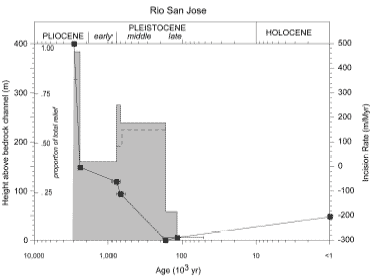
Lava flows can preserve the landscape onto which they are erupted and geologists often use the difference in the elevation of drainages preserved under lava flows compared to the elevation of modern drainages to measure the amount and rate of erosion since volcanism began. Lipman and Mehnert (1979) noted that drainage levels have dropped on average 820 to 980 ft (250 to 300 m) during the last ~3 million years (Figure 4). Love (1989) and Love and Connell (2005) have determined that the Rio San Jose drainage system south of Mt. Taylor has had a relatively complex incision history related in part to recurring volcanism in the area, change in base level in the Rio Grande system, and climate. Love (1989) note that the total relief between the base of the lava flows on Horace Mesa (Figure 3) to the base of an ancient valley floor buried by modern alluvium is on the order of 1312 ft. (400 m) (Figure 6). Love and Connell (2005) document 823 ft (251 m) of downcutting between 2.93 and 2.39 million years ago (~1509 ft/m.y. or 460 m/m.y.!) and 489 ft (149 m) of downcutting between 2.39 million and 170 thousand years ago (~223 ft/m.y. or 68 m/m.y.) (Figure 7). Since 170,000 years ago, erosion in the drainage has ceased and 161 ft (49 m) of alluvium has accumulated.
References
- Aubele, J.C., Crumpler, L.S., Loeber, K.N., and Kudo, A.M., 1976, Maars and tuff rings of New Mexico: New Mexico Geological Society Special Publication 6, p. 109-114
- Baker, I., and Ridley, W.I., 1970, Field evidence and K, Rb, and Sr data bearing on the origin of the Mt. Taylor volcanic field, New Mexico, U.S.A., Earth and Planetary Science Letters, v. 10, no. 1, p. 106-114.
- Baldridge, W.S., Perry, F.V., Vaniman, D.T., Nealey, L.D., Leavy, B.D., Laughlin, A.W., Kyle, P. , Bartov, Y., Steinitz, G., and Gladney, E.S., 1989, Magmatism associated with lithospheric extension: Middle to late Cenozoic magmatism of the southeastern Colorado Plateau and central Rio Grande rift, New Mexico and Arizona: New Mexico Bureau of Mines and Mineral Resources Memoir 46, p. 187-202.
- Bassett, W.A., Kerr P.F., Schaeffer, O.A., and Stoenner, R.W., 1963, Potassium-argon ages of volcanic rocks near Grants, New Mexico: Geological Society of America Bulletin, v. 74, p. 221-226.
- Cather, S.M., 2004, The Laramide orogeny in central and northern New Mexico and southern Colorado, in Mack, G.H., and Giles, K.A., eds., The Geology of New Mexico, A Geologic History: New Mexico Geological Society Special Publication 11, p. 203-248
- Crumpler, L.S., 1977, Alkali basalt-trachyte suite and volcanism, north part of the Mount Taylor volcanic field, New Mexico: M.S. Thesis, University of New Mexico, Albuquerque, 131 pp.
- Crumpler, L.S., 1980, An alkali-basalt through trachyte suite, Mesa Chivato, Mount Taylor volcanic filed, New Mexico: Geological Society of America Bulletin, Part II, v. 91, p. 1293-1331.
- Crumpler, L.S., 1982, Volcanism in the Mount Taylor region: New Mexico Geological Society Guidebook 33, p. 291-298.
- Dillinger, J.K., 1990, Geologic map of the Grants 30' x 60' quadrangle, west-central New Mexico: U.S. Geological Survey Coal Investigations map C-118-A, scale 1:100,000.
- Dunbar, N.W., and Phillips, F.M., 2004, Cosmogenic 36Cl ages of lava flows in the Zuni–Bandera volcanic field, northcentral New Mexico, U.S.A.: New Mexico Bureau of Geology and Mineral Resources, Bulletin 160, p. 309-317.
- Frey, Bonnie A., Kelley, Shari A., Zeigler, Kate E., McLemore, Virginia T., Goff, Fraser, Ulmer-Scholle, Dana S., 2021, Geology of the Mount Taylor area, New Mexico Geological Society, Guidebook, v. 71, 310p. ISBN: 1-58546-112-1.
- Goff, F., Kelley, S.A., Goff, C.J., McCraw, D.J., Osburn, G.R., Lawrence, J.R., Drakos, P.G., and Skotnicki, S.J., 2019, Geologic map of the Mount Taylor volcano area, New Mexico: New Mexico Bureau of Geology and Mineral Resources, Geologic Map 80, 1:36,000 scale w/ 57 page booklet.
- Hunt, C.B., 1938, Igneous geology and structure of the Mount Taylor volcanic field, New Mexico: U.S. Geological Survey Professional Paper 189-B, p. 51-80.
- Keating, G.N., and Valentine, G.A., 1998, Proximal stratigraphy and syn-eruptive faulting in rhyolitic Grants Ridge Tuff, New Mexico, USA: Journal of Volcanology and Geothermal Research, v. 81, p. 37-49.
- Kerr, P.F., and Wilcox, J.T., 1963, Structure and volcanism, Grants Ridge area, New Mexico, in Geology and Technology of the Grants Uranium Region: New Mexico Bureau of Mines and Mineral Resources Memoir 15, p. 205-213.
- Laughlin, A.W., Brookins, D.G., and Damon, P.E., 1976, Late Cenozoic basaltic volcanism along the Jemez zone of New Mexico and Arizona: Geological Society of America Abstracts with Programs: v. 8 p. 1976.
- Laughlin, A.W., Perry, F.V., Damon, P.E., Shafiqullah, M., WoldeGabriel, McIntosh, W.C., Harrington, C.D., Wells, S.G., and Drake, P.G., 1994, Geochronology of the Mount Taylor, Cebollita Mesa, and Zuni-Bandera volcanic fields, Cibola County, New Mexico: New Mexico Geology, v. 15, p. 81-92.
- Lipman, P.W. And Mehnert, H.H., 1979, Potassium-argon ages from the Mount Taylor Volcanic Field, New Mexico: U.S. Geological Survey Professional Paper 1124-B, 8 pp.
- Lipman P.W., and Moench, R.H., 1972, Basalts of the Mount Taylor volcanic field, New Mexico: Geological Society of America Bulletin, v. 83, no. 5, p. 1335-1343.
- Lipman, P.W., Pallister, J.S., and Sargent, K.A., 1979, Geologic map of the Mount Taylor quadrangle, Valencia County, New Mexico: U.S. Geological Survey Map, GQ-1523: scale 1:24,000.
- Love, D.W., 1989, Geomorphic development of the Rio Puerco Valley, New Mexico Geological Society Guidebook 40, p. 11-12.
- Love, D.W., and Connell, S.D., 2005, Late Neogene drainage development on the southeastern Colorado Plateau, New Mexico: New Mexico Museum of Natural History and Science Bulletin 28, p. 151-169.
- Mayo, E.B., 1958, Lineament tectonics and some ore districts of the southwest, Transactions of AIME, v. 211, 1169-1175
- Moench,R.H., and Schlee, J.S., 1967, Geology and uranium deposits of the Laguna district, New Mexico: U.S. Geological Survey Professional Paper 519, 117 pp.
- Thaden, R.E., Santos, E.S., and Raup, O.B., 1967, Geologic map of the Grants Quadrangle, Valencia county, New Mexico: U.S. Geological Survey Map GQ-681, scale 1:24,000.
- Perry, F.V., Baldridge, W.S., DePaolo, J., and Shafiqullah, M., 1990, Evolution of a magmatic system during continental extension: the Mount Taylor volcanic field, New Mexico: Journal of Geophysical Research, v. 95, p. 19327-19348.
- Porreca, C., Selverstone, J., and Brearly, A., 2004, A partial transformation of garnet lhersolite to spinel websretie in henoliths from the Rio Puerco volcanic field, New Mexico: A record of incipient extension, Geological Society of America Abstracts with Programs, v. 36.
- WoldeGabriel, G., Keating, G.N., and Valentine, G.A., 1999, Effects of shallow basaltic intrusion into pyroclastic deposits, Grants Ridge, New Mexico, USA, Journal of Volcanology and Geothermal Research, v. 92, p. 389-411.