
Tyrone Mine
Introduction
The Tyrone mining district, the second largest porphyry copper deposit in New Mexico, is located 10 miles southwest of Silver City in the Burro Mountains of southwestern New Mexico (Figure 1; McLemore, 2008). Native Americans initially mined turquoise from the area around 600 AD (McLemore, 2008). Underground mining was established in the early 1860s and the current mode of mining, open-pit stripping, began in 1968. The mine became part of Freeport-McMoRan in 2007. Parts of the pit are currently being reclaimed (Figure 2; McLemore, 2008).
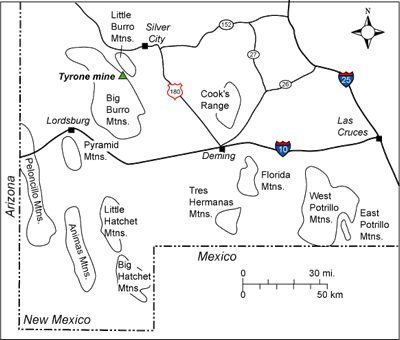
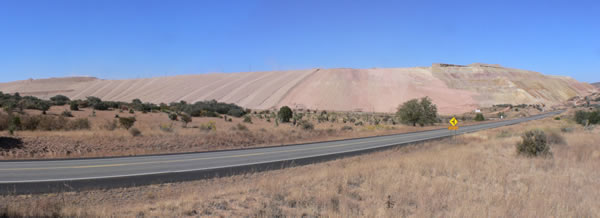
Regional Setting
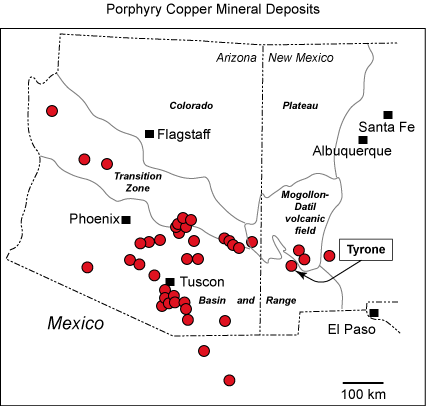
The Tyrone mine is in the Basin and Range province along the southern margin of the Mogollon-Datil volcanic field. The Mogollon-Datil volcanic field straddles the transitional boundary between the tectonically stable Colorado Plateau to the north and the extensional terrane of the Basin and Range to the south (Figure 3).
The mining district is in the northwest-striking Burro Mountain uplift. The northwest-trending structural grain in southwestern New Mexico is primarily the result of Jurassic rifting and subsequent Laramide compressional deformation. The mineralization is in and around the Tyrone stock, a 53 to 57 million-year-old quartz monzonite porphyry (DuHamel et al., 1995) that is just one of many intrusions emplaced across southern Arizona and southwestern New Mexico during Laramide deformation 80 to 40 million years ago (Figure 3).
Geologic History
The oldest rocks in the Burro Mountain uplift are Proterozoic metamorphic rocks, including schist, gneiss, and amphibolite. The schist was originally shale or calcareous sediment, the gneiss may have been arkose and the amphibolite was originally basalt (Hedlund, 1980; Sanders, 2003; Amato et al., 2008a). These ancient sedimentary and volcanic rocks were buried and heated during an episode of mountain building that affected southwestern New Mexico 1.6 to 1.7 billion years ago (Amato et al., 2008a). Starting about 1.46 billion years ago, the metamorphic rocks were intruded by several granitic plutons of variable texture and composition (Amato et al., 2008b). Mineralization associated with contact metamorphism around pegmatites in these younger Proterozoic intrusions include rare-earth elements, magnetite, and fluorite (Gillerman, 1970). Later in Proterozoic time, NW-striking fractures formed and the fractures filled with mafic dikes (Gillerman, 1970).
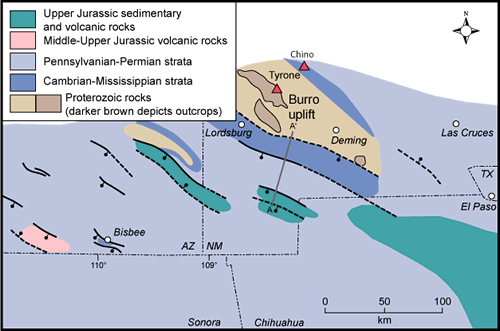
The geologic history of the Burro Mountain region between Proterozoic time and Cretaceous time is poorly known. The Paleozoic strata preserved in the Cobre Mountains near the Chino Mine to the northeast were eroded from the Burro Mountains prior to Cretaceous time. The Burro Mountains were a highland on the flank of a northwest-trending late Jurassic to early Cretaceous rift basin known as the Bisbee Basin (Figure 4); thus the older portion of the Mesozoic section was either never deposited or was eroded. Cretaceous rocks lapped onto and buried the rift shoulder (Figure 5). Upper Cretaceous Beartooth Quartzite unconformably overlies Proterozoic rocks in the Little Burro Mountains (Figure 6). The Beartooth Quartzite is gradationally overlain by the Colorado Formation, which consists of a shale member and a sandstone member. The Upper Cretaceous rocks preserve a history of the oscillating landward and seaward migration of the shoreline of the Western Interior Seaway across this area about 90 to 105 million years ago.
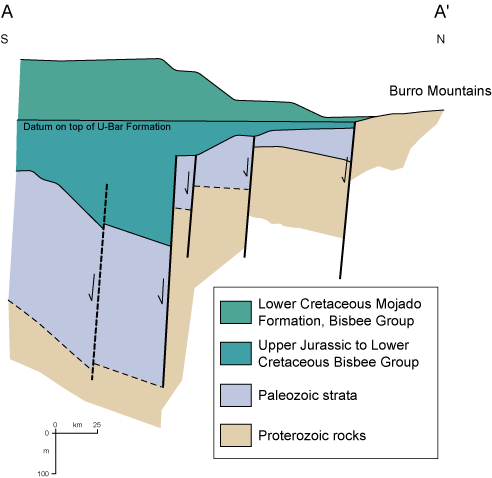
Rhyolite plugs, dikes, and ENE-trending fractures formed prior to the emplacement of the Tyrone stock (Gillerman, 1970). The Cretaceous and older rocks were subsequently invaded by the Tyrone stock 53 to 57 million years ago, coincident with compressive Laramide deformation in this region. Many of the NW-trending normal faults associated with Jurassic rifting where reactivated as reverse faults during Laramide deformation (Lawton, 2000). Northeast- to east-striking normal faults developed in the Burro Mountains during Laramide deformation after the emplacement of the Tyrone stock (Gillerman, 1970; Figure 6).
What triggered all the volcanic activity in southwestern New Mexico? The activity can be explained in terms of plate tectonics. According to one hypothesis, the story of the porphyry copper deposit and Mogollon-Datil volcanic field actually began nearly 100 million years ago and hundreds of miles to the west, when the Farallon Plate was subducted eastward under the western edge of the North American Plate (Atwater, 1970; Lipman et al., 1972; Elston, 1976). This plate, which was between the Pacific and the North American plates, has largely been consumed by subduction during the last 100 million years; we now see only two small fragments of it on the ocean floor off the west coast today. Originally, the Farallon Plate was subducted beneath North America at a fairly steep angle, giving rise to the magmatism preserved in the roots of the Sierra Nevada in California and the coastal range batholiths of California and Baja California. Magmatism migrated eastward and arrived in southwestern New Mexico approximately 70 million years ago. This eastward migration is thought to have been caused by flattening of the subduction angle of the Farallon plate, initiated either by an increase in the rate of subduction or by subduction of a buoyant oceanic plateau. A similar situation can be observed today off the west coast of South America (e.g., Allmendinger et al., 1997). Between about 70 and 45 million years ago, the amount of magmatism dramatically decreased because the slab was subducting at such a shallow angle that little melting could occur. However, some magmatism did occur in southwestern New Mexico during this time, as indicated by all the activity in the Silver City region. McMillan (2004) postulates that the lithosphere under the Jurassic to early Cretaceous Bisbee Basin rift was still warm 70 to 45 million years ago and facilitated Laramide plutonism in this region. Compression of the crust associated with low-angle subduction led to the formation of northwest-trending mountain ranges in southwestern New Mexico.
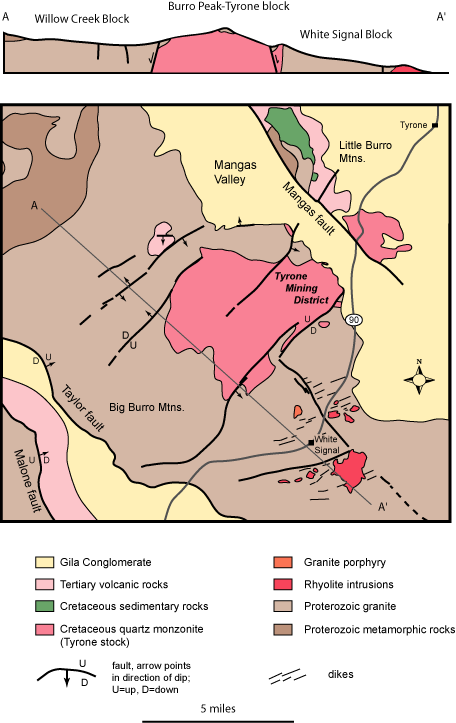
Between 45 and 36 million years ago, the subduction of the Farallon Plate slowed and the angle of subduction started to increase. New Mexico was still under mild compression as early andesites in the Mogollon-Datil volcanic field began to erupt (Cather, 1990; McIntosh et al., 1991; Chapin et al., 2004). As the slab continued to roll back or sink down into the mantle, hot asthenosphere came in contact with lithospheric mantle that had been enriched by fluids during the subduction process. In addition, the crust began to extend. As a consequence, the conditions were right for voluminous caldera-forming eruptions in Mogollon-Datil field 36 to 24 million years ago. The 33.5 million-year-old Box Canyon Tuff, the 27.6 million-year-old tuff of Walking-X Canyon, and other tuffs and lava flows covered the Burro Mountains (McIntosh et al., 1991; Chapin et al., 2004).
Extension along the northwest-striking faults that define the margins of the Little and Big Burro Mountains (Figure 6) began during Miocene time. Sediments eroded from the rising mountains were deposited in the Gila Conglomerate in the valleys. The faults on the southwest side of the mining district, the northeast-dipping Taylor and Malone faults (Figure 6), form a complex graben. The fault on the southwest side of the Little Burro Mountains is the southwest-dipping Mangas fault. These mountain-bounding faults cut the Pliocene Gila Conglomerate, indicating that these faults were active less than 5 million years ago. In addition, the NE-striking Burro Chief fault in the Tyrone stock cuts the Gila Conglomerate (Figure 6).
Mineralization
Porphyry copper deposits are low-grade (<0.8%) disseminated deposits of copper found in and around small intrusive bodies composed of porhyritic diorite, granodiorite, monzonite or quartz monzonite (McLemore, 2008). The small plutons (also called stocks) are often shallowly emplaced at depth within 1 to 6 km of the earth's surface. The copper occurs within breccia or in networks of fractures, both in the porphyritic intrusion and in the adjoining country rocks (Figure 7). Steam and gas concentrated during the final phases of the cooling of a magma body becomes pressurized, fracturing the solidified portions of the pluton and the surrounding country rock. The mineralized fluids fill fractures, cool, and precipitate minerals such as copper. Other economic minerals deposited by this process include gold, silver, molybdenum, and uranium. The mineral pyrite, while not economic, is pervasively deposited as well. Because there is a gradation in temperature from hot to less hot away from the pluton and mineral formation is often sensitive to temperature, the type of mineral deposited is commonly concentrically zoned around an intrusion. Primary mineralization of the upper part of the Tyrone stock and the surrounding Proterozoic country rock occurred during the late phases of cooling of the Tyrone quartz monzonite porphyry.
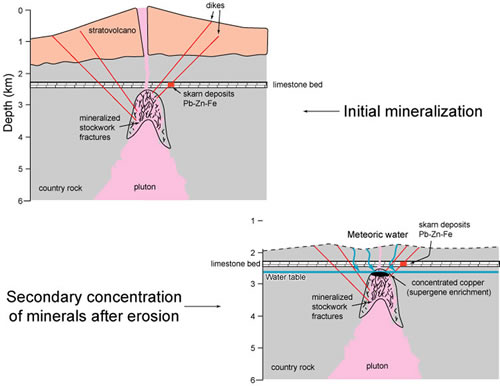
The initial copper mineralization of the Tyrone stock was low grade and not economic. Later, NE-striking faults and fractures formed, creating a central upthrown block or horst called the Tyrone-Burro Peak block that formed a structural high relative to areas to the southeast (White Signal block) and to the northwest (Willow Creek block; Figure 6). The mineralized rocks were brought toward the surface by uplift and erosion, where the rocks interacted with groundwater. The copper was concentrated later during weathering of the stock near the earth's surface (Figure 7). Oxygenated water seeped from the surface into the mineralized country rock and stock that contained abundant pyrite, as well as disseminated minerals of economic interest. The water interacted with the pyrite (FeS2) to form sulfuric acid. The acid leached copper-bearing minerals from the rocks, causing the copper to go into solution. When this acidic, oxidized, copper-enriched solution hit the water table, the chemistry of the solution changed so that it was no longer acidic or oxygenated. The copper came out of solution, replacing chalcopyrite and pyrite deposited in the rock during the initial mineralization with the copper mineral, chalcocite (Cu2S), and to a lesser extent, covellite, thus forming a zone of enriched copper mineralization. This natural process is called supergene enrichment (Figure 7). Mineralization is concentrated along the NE-striking structures in the Tyrone mining district. The initial supergene enrichment at Tyrone occurred 44 to 47 million years ago (DuHamel et al., 1995; Cook, 1994).
Tertiary volcanism led to a younger episode of mineralization in the Tyrone mining district that deposited fluorite, gold and uranium minerals (Kolessar, 1970). Tilting during more recent Basin and Range deformation caused part of the supergene ore at Tyrone to be exposed and oxidized, forming chrysocolla and other oxidized copper minerals. A second phase of supergene enrichment occurred 19 million years ago (DuHamel et al., 1995; Cook, 1994).
References
- Allmendinger, R. W., Jordan, T. E., Kay, S. M., and Isacks, B. L., 1997, The evolution of the Altiplano–Puna Plateau of the central Andes: Annual Reviews of Earth and Planetary Sciences, v. 25, pp. 139–174.
- Amato, J. M., Boullion, A. O., Serna, A. M., Sanders, A. E., Farmer, G. L., Gehrels, G. E., and Wooden, J. L., 2008a, The evolution of the Mazatzal province and the timing of the Mazatzal orogeny: Insights from U-Pb geochronology and geochemistry of igneous and metasedimentary rocks in southern New Mexico: Geological Society of America Bulletin, v. 120, p. 328-346, doi: 10.1130/B26200.1.
- Amato, J., Boullion, A.O., and Sanders, A.E., 2008b, Magmatism and metamorphism at 1.46 Ga in the Burro Mountains, southwestern New Mexico: New Mexico Geological Society Guidebook, 59th Fall Field Conference, p. 107-116.
- Atwater, T., 1970, Implications of plate tectonics for the Cenozoic tectonic evolution of western North America: Geological Society of America Bulletin, v. 81, no. 12, pp. 3513–3536.
- Burnham, C.W., 1979, Magmas and hydrothermal fluids, in Barnes, H.L., ed., Geochemistry of hydrothermal ore deposits (2d ed.): New York, John Wiley & Sons, Inc., p. 71–136.
- Cather, S. M., 1990, Stress and volcanism in the northern Mogollon–Datil volcanic field, New Mexico—effects of the post-Laramide tectonic transition: Geological Society of America Bulletin, v. 102, no. 11, pp. 1447–1458.
- Chapin, C.E., McIntosh, W.C., and Chamberlin, R.M., 2004, The Late Eocene—Oligocene peak of Cenozoic volcanism in southwestern New Mexico, in Mack, G.H., and Giles, K.A., The Geology of New Mexico, a Geologic History: New Mexico Geological Society Special Publication 11, 271-294.
- Cook, S.S., 1994, The geologic history of supergene enrichment in the porphyry copper deposits of southwestern North America [Ph.D. dissertation]: University of Arizona, Tucson, 163 pp.
- DuHamel, J.E., Cook, S.S., and Kolessar, J., 1995, Geology of the Tyrone porphyry-copper deposit, New Mexico, in Pierce, F.W., and Bolm, J.G., eds., Porphyry-Copper Deposits of the American Cordillera: Arizona Geological Society Digest, v.20, p. 464-472.
- Elston, W. E., 1976, Tectonic significance of mid-Tertiary volcanism in the Basin and Range province—a critical review with special reference to New Mexico; in Elston, W. E., and Northrop, S. A. (eds.), Cenozoic volcanism in southwestern New Mexico: New Mexico Geological Society, Special Publication 5, pp. 93–102.
- Gillerman, E., 1970, Mineral deposits and structural pattern of the Big Burro Mountain, New Mexico: New Mexico Geological Society Guidebook, 21st Fall Field Conference, p. 115-121.
- Hedlund, D.C., 1980, Geologic map of the Redrock NW quadrangle, Grant County, New Mexico: Reston, Virginia, U.S. Geological Survey Miscellaneous Field Studies, Map MF-1263, scale 1:24,000.
- Humphreys, E., Hessler, E., Dueker, K., Farmer, C., Erslev, E., and Atwater, T., 2003, How Laramide-age hydration of North American lithosphere by the Farallon slab controlled subsequent activity in the western United States: International Geology Review, v. 45, p. 575–595.
- Kolessar, J., 1970, Geology and copper deposits of the Tyrone district: New Mexico Geological Society Guidebook, 21st Fall Field Conference, p. 127-132.
- Lawton, T.F., 2000, Inversion of Late Jurassic-Early Cretaceous extensional faults of the Bisbee Basin, southeastern Arizona and southwestern New Mexico: New Mexico Geological Society Guidebook, 51st Fall Field Conference, p. 95-102.
- Lipman, P. W., Protska, J. J., and Christiansen, R. L., 1972, Cenozoic volcanism and plate tectonic evolution of the western United States, I, Early and middle Cenozoic: Royal Society of London, Philosophical Transactions, Series A, v. 271, no. 1213, pp. 217–248.
- McIntosh, W.C., Kedzie, L.L., and Sutter, J.F., 1991, Paleomagnetism and 40Ar/39Ar ages of ignimbrites, Mogollon-Datil volcanic field, southwestern New Mexico: New Mexico Bureau of Geology and Mineral Resources Bulletin 135, 79 pp.
- McLemore, V.T., 2008, Tyrone mine, Burro Mountains district, Grant County, New Mexico: New Mexico Geological Society Guidebook, 59th Fall Field Conference, p. 54-56.
- McMillan, N.J., 2004, Magmatic record of Laramide subduction and the transition to Tertiary extension: upper Cretaceous through Eocene igneous rocks of New Mexico, in Mack, G.H., and Giles, K.A., The Geology of New Mexico, a Geologic History: New Mexico Geological Society Special Publication 11, p. 249-270.
- Sanders, A.O., 2003, Age of deposition and metamorphism of deformed Proterozoic metasedimentary rocks in the Burro Mountains, southwest New Mexico [M.S. thesis]: Las Cruces, New Mexico, New Mexico State University, 198 p.
- Titley S. R. and Anthony E. Y., 1989, Laramide mineral deposits in Arizona, in Jenney J. P. and Reynolds S. J. eds., Geologic Evolution of Arizona: Arizona Geological Society Digest, v. 17, p.485–514.
- Zientek, M.L. and Orris, G.J., 2005, Geology and nonfuel mineral deposits of the United States, U.S. Geological Survey Open-File Report 2005-1294A, 179 pp.