
Caballo Lake & Percha Dam State Parks
Location
Caballo Lake & Percha Dam State Parks are located approximately 14 miles south of the town of Truth or Consequences. Caballo Reservoir is on the Rio Grande in south-central New Mexico east of Interstate 25 between Socorro and Las Cruces (Figure 1).
Camping, picnicing, and boating facilities are available at both of these state parks. Caballo Lake State Park is the third largest state park in New Mexico. This park is generally quiet compared to Elephant Butte State Park to the north.
Introduction
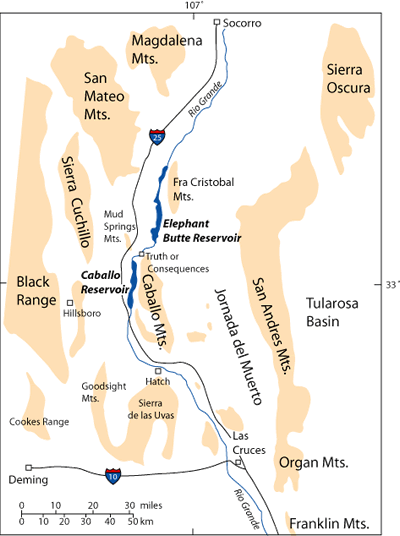
The dam across the Rio Grande that created Caballo Reservoir was completed in 1938 approximately 22 miles (35 km) downstream from Elephant Butte Dam, which was built between 1912 and 1916 (Mueller, 1986). Caballo Dam was built to control water releases from Elephant Butte Dam. Both Caballo and Elephant Butte Reservoirs are integral parts of the water storage system along the Rio Grande and its tributaries needed to deliver a relatively predictable water supply to southern New Mexico, West Texas, and Mexico. A power plant, originally designed to provide electricity year-around, was added to the Elephant Butte Dam in 1940 (Mueller, 1986). Water released during power generation at Elephant Butte Dam is stored downstream in Caballo Reservoir. Elephant Butte Dam now generates electricity primarily during the irrigation season (mid-March to mid-October). Any water released from Elephant Butte Dam in the winter for power generation is stored at Caballo Dam for irrigation use in the summer.

Caballo Dam is an earth fill dam that is 96 feet high and 4558 feet long (Figure 2). Although Caballo Reservoir is shallow (maximum depth of 25 feet), it covers a large area. The greatest storage (346,570 acre-ft.)occurred in March of 1942, when the water level was less than an inch above flood pool elevation. The lake can now hold 343,000 acre-feet of water. The original intended capacity was 344,000 acre-feet, but sedimentation over the years is gradually reducing the lake's storage capacity. An acre-foot is equivalent to 325,829 gallons.
Regional Geologic Setting
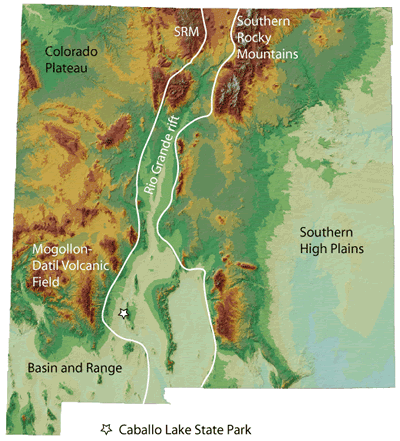
Caballo Lake State Park is in the Rio Grande rift, a northerly-trending continental rift that extends from northern Colorado to northern Mexico (Figure 3). The park is in a portion of New Mexico that has been affected by compression, regional volcanism, and extension during the last 70 million years (Kelley and Silver, 1952: Chapin and Seager, 1975; Seager et al., 1984; Seager and Mack, 1986; Mack and Seager, 1990; Mack et al., 1994 a,b, and Seager and Mack, 2003). First, Laramide compression associated with a subduction zone along the west coast of North America formed west-northwest trending basement uplifts bounded by southwest-dipping, high-angle reverse faults (Seager and Mack, 1986; Seager et al., 1986). This compressional phase occurred mainly in the Paleocene to early Eocene (~55 to 45 million years ago), although there is evidence for deformation as early as late Cretaceous and as late as late Eocene. Following Laramide deformation, regional latest Eocene to Oligocene (36 to 27 million years ago) volcanism from the Mogollon-Datil volcanic field and other volcanic centers blanketed large portions of southern New Mexico with layers of ash-flow tuff and basalt flows. The distribution of tuff indicates that topographic relief during middle Oligocene time was not as large as it is today. Volcanism was followed by, and was in part coeval with, extension starting about 36 million years ago, leading to the development of the Rio Grande rift.
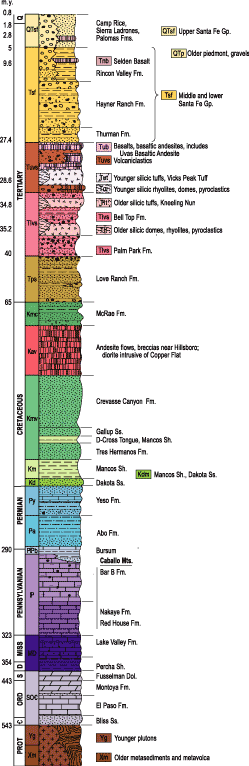
Brief Geologic History
Remnants of an extensive latest Eocene to Miocene volcanic complex, the Mogollon-Datil volcanic field, form the distant hills to the west of the park. The rugged Caballo Mountains, with an average local relief of about 2000 feet (610 m), dominate the scenery to the east of the state park (Figure 5). The Caballo Mountains are an east-dipping fault block with Proterozoic granitic and metamorphic rocks exposed on the rugged western side. Paleozoic to Mesozoic sedimentary rocks overlie the Proterozoic rocks and form the eastern dip slope into the Jornada del Muerto basin (Figure 1). Paleozoic sedimentary rocks that form the crest of the range are dominated by limestone and dolomite. These mountains are exceptional in southern New Mexico because 3.5 miles (5.6 km) of stratigraphic section representing all time periods except the Triassic and Jurassic are preserved in the rock record here (Kelley and Silver, 1952: Seager and Mack, 2003; Figure 4). A rich record of four episodes of mountain building, five major cycles of the rise and fall of warm tropical seas, and the remnants of distant volcanic eruptions is found in this remarkable place.
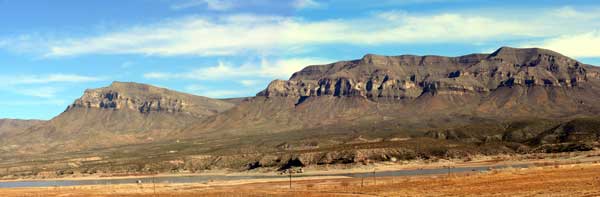
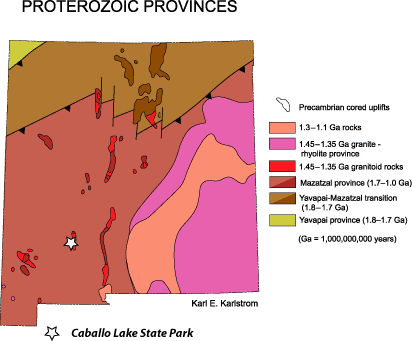
The oldest rocks in the Caballo Mountains are Proterozoic metavolcanic and metasedimentary rocks, which are preserved in a few places along the west side of the mountain range. These rocks originally were volcanic rocks, sandstone, and shale deposited in an extensional basin about 1.65 to 1.60 billion years ago. These volcanic and sedimentary rocks were buried, deformed, and heated during a mountain building event about 1.6 billion years ago. The combination of high temperatures and high pressures at great depth in the Earth transformed the volcanic and sedimentary rocks into metavolcanic and metasedimentary rocks. Later, granite that is estimated to be 1.4 billion years old intruded into the older metamorphic rocks that had formed in the roots of this ancient northeast-trending ~1.6 billion year-old mountain belt (Figure 6). Syenite of possible Cambrian age intrudes the granite (McLemore, 1986).
After a long period of erosion, a shallow ocean covered the Caballo Mountain region starting about 510 million years ago. During early to middle Paleozoic time, southern New Mexico was located just south of the equator (Figure 7). Shallow seas advanced and retreated across the area several times between 510 and 340 million years ago, depositing limestone, dolomite, shale and sandstone, until the second mountain building event, Ancestral Rockies deformation, began.
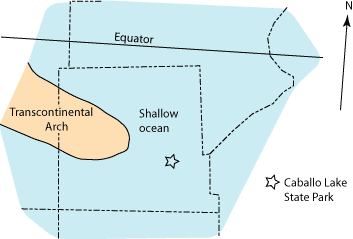
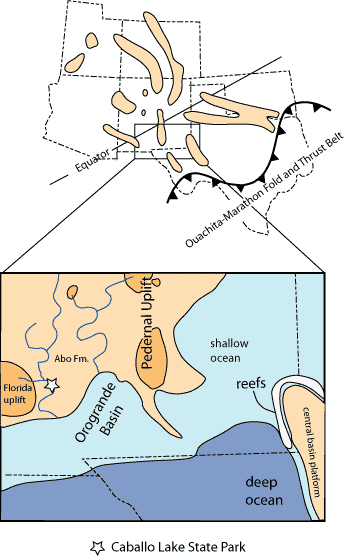
Ancestral Rocky Mountain development across the southwestern United States is characterized by north- and northwest-trending uplifts separated by intervening basins that are filled with both marine sediments and sediments shed from the adjacent uplifts (Figure 8). The Caballo Mountains were located on the western margin of one of the basins, the Orogrande Basin, so tectonism in this area related to this event was relatively mild. Rocks older than early Mississippian in age (>340 million years old) in the Caballo Mountains were tilted toward the southeast and eroded prior to deposition of early Pennsylvanian sediments about 312 million years ago. In the early stages of this tectonic episode, shallow seas persisted in the Caballo Mountain region until ~290 million years ago, when sea level dropped and rivers started to flow southward across the area. Windblown sand dune deposits marked a significant drying trend in New Mexico starting ~270 to 280 million years ago. The margin of the ocean returned late in Permian time and moved back and forth across the area, depositing limestone, dolomite, gypsum, and sandstone.
No deposition occurred in the Caballo Mountain region during most of Mesozoic time because southwestern New Mexico, including the area of interest, was on the northeastern flank of a west-northwest-trending rift that was active into Middle Cretaceous time. Upper Cretaceous rocks lapped onto and buried the rift shoulder. The Upper Cretaceous rocks preserve a history of the oscillating landward and seaward migration of the shoreline of the Western Interior Seaway across this area about 95 to 80 million years ago. Following retreat of the sea, the Caballo Mountains were apparently covered with andesitic lavas from a stratovolcano located to the west of the range that has since been buried beneath the Rio Grande rift. The andesitic debris was eroded from the Caballo Mountains and deposited as conglomerates in the latest Cretaceous McCrae Formation, which crops out on the east side of the range. This interesting unit also contains siliceous tuffs, fossil leaves, petrified wood, and dinosaur bones. The distribution of the McCrae Formation in the Caballo Mountains records the beginning of the third mountain building event in the Caballo Mountains, compressional Laramide deformation. Laramide deformation reached its peak during Eocene time, forming a northwest-trending uplift in the southern Caballo Mountains and a basin in the northeastern Caballo Mountains (Figure 9). Following Laramide deformation, sediments shed from andesite volcanoes covered the area.
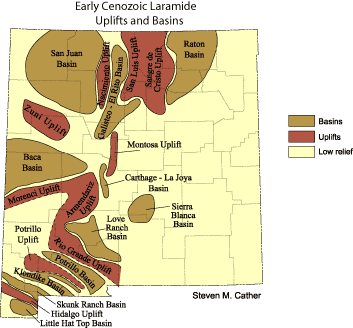
Starting about 36 million years ago caldera eruptions in areas to the west and northwest of the Caballo Mountains blanketed the area with rhyolitic tuff and tuffacous volcaniclastic sediments, while basaltic andesite from shield volcanoes and fissure eruptive centers to the southwest (Cedar Hills vent zone, Figure 10) and east (Point of Rocks) of the range flowed across the region. Minor faulting accompanied regional volcanism. The Caballo Mountains began to assume their final shape starting about 25 million years ago, with the current mountain block rising about 5 million years ago along a major fault on the west side of the range.
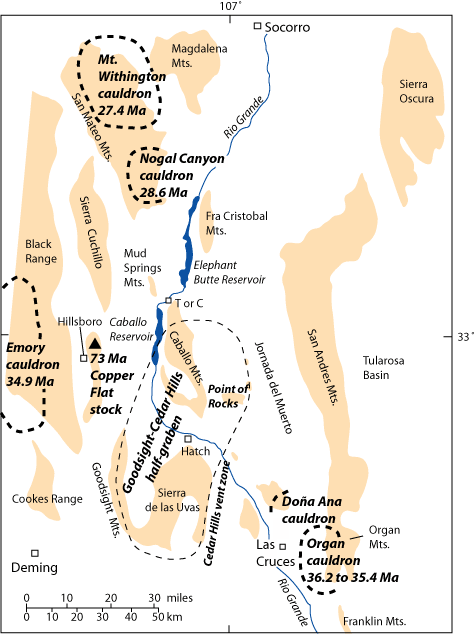
A More In-Depth Geologic History
Proterozoic
The roots of the earliest mountain ranges in south-central New Mexico, which generally trended northeast (Condie and Budding, 1979; Karlstrom et al., 2004), are preserved in the Proterozoic amphibolite, schist, and gneiss exposed on the west side of the Caballo Mountains. The original rocks prior to metamophism were basaltic to rhyolitic volcanic and shallow intrusive rocks interbedded with sandstone and shale that were likely deposited in an extensional basin. Although no age dates are available for the oldest rocks in the Caballo Mountains, they are probably 1.6 to 1.65 billion years old, based on the ages of similar rock types found elsewhere in New Mexico (Condie and Budding, 1979, Bauer and Lozinsky, 1986; Karlstrom et al., 2004). As the basin closed and this portion of New Mexico became part of the North American continent, the rocks were folded and heated to become amphibolite-grade metamorphic rocks in the root of an ancient mountain range. The metamorphic rocks were later intruded by 1.4(?) billion year old granites are also foliatited, which means that lateral deformation related to mountain building continued during the intrusive events.
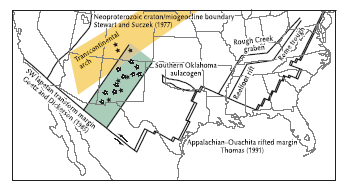
Several small bodies of a brick-red igneous rock known as syenite intrude the Proterozoic granites in the southern Caballo Mountains. No age dates are available, but McLemore (1986) speculates that these intrusions are Cambrian in age, based on the similarity of these rocks to dated Cambrian intrusions in other parts of New Mexico. These bodies, along with other Cambrian syenite intrusions in New Mexico and southern Colorado may have been emplaced in a failed north-northeast-trending rift (McMillan and McLemore, 2004: Figure 11).
Early to Middle Paleozoic
Early to Middle Paleozoic sandstone, limestone, and dolomite deposited in transgressing and regressing shallow, equatorial seas rest on an erosion surface cut on the Proterozoic rocks. The oldest of these units, the latest Cambrian to earliest Ordovician Bliss Formation, has a basal conglomerate with quartz, metamorphic, and granite clasts derived from the underlying basement overlain by brown-tan arkosic sandstone and a thin bed of red oolitic ironstone. The upper part of the Bliss Formation consists of green siltstone and gray-brown limestone. Burrows and brachiopod, trilobite, and echinoderm fragments are common in this unit. The Bliss Formation was deposited during a east-northeast transgression of the ocean in a near shore environment.
The Bliss Formation grades up into the limestone and dolomite of the Early Ordovician El Paso Formation. Burrows, as well as fragments of trilobites, echinoderms, gastropods, cephalopods, sponges, and Nuia (algae), are frequently found in the limestone (Clemons, 1984, 1991). A stomatolite bed occurs near the top of the unit (Seagar and Mack, 2003). The marine transgression that began in late Cambrian time continued into the time of El Paso Formation carbonate deposition, with the carbonates of the El Paso Formation forming below storm wave base in a sea deeper than that of Bliss time. A regional drop in sea level is indicated by the stromatolite bed that was deposited near the end of Early Ordovician time.
The dark brown Cable Canyon sandstone member, the basal unit of the Late Middle to Late Ordovician Montoya Formation, was deposited on an erosion surface cut into the top of the Early Ordovician El Paso Formation. The remainder of the Montoya Formation consists of fossiliferous cherty dolomite and dolomite, indicating a rise in sea level following deposition of the Cable Canyon sandstone. The Cable Canyon member is interpreted to have been deposited as sand ridges that formed slightly offshore in a shallow sea (Bruno and Chafetz,1988), while the carbonates in the lower to middle part of the unit were deposited further offshore on a ramp covered by shallow, warm ocean water that became cooler and deeper with time (Pope, 2002). Fossils in the carbonates include corals, echinoderms, brachiopods, and bryozoans. The chert nodules contain abundant sponge spicules. The uppermost carbonates in the unit were deposited in shallow water, perhaps in a lagoonal or tidal setting (Pope, 2002).
A small gap in the rock record caused by erosion or nondeposition occurs between the Montoya Formation and the overlying Fusselman Dolomite. The Middle Silurian Fusselman Dolomite consists of a basal cross-bedded sandy dolomite and a gray, medium-bedded cherty dolomite above. A few fragmentary horn corals and crinoid stems are preserved in the basal section. Dolomitization has erased many of the sedimentary structures, so the depositional environment for the Fusselman Dolomite is unclear, although it is most likely shallow marine.
Another gap in the rock record exists between Late Silurian and Late Devonian time. A marine transgression deposited the greenish-gray to black Late Devonian Percha Shale. The shale, which contains only a few brachiopods, is interbedded with thin siltstone and limestone beds. The shales suggest deposition in quiet, oxygen-poor, marine water. The unit grades up into the carbonates of the overlying Mississippian Lake Valley Formation. The bluish gray limestone, dolomite, and cherty limestone of the Lake Valley Formation was deposited on a south-dipping marine ramp during the transgression that began in Late Devonian time. This unit is famous for well-preserved fossils that include brachiopods, echinoderms, horn coral, and bryozoans. Towards the end of Lake Valley sedimentation, the Lake Valley Formation was deposited in shallower water, signaling both a drop in sea level and tectonic tilting of the early Mississippian and older rocks toward the southeast. The tilted rocks were eroded prior to the deposition of the early Pennsylvanian Magdalena Group, forming an angular unconformity that developed over an interval of about 30 million years.
Late Paleozoic
The Pennsylvanian to early Permian Magdalena Group (= Madera Group of Kues (2001)), which is divided into the Red House, Nakaye, and Bar B formations by Seager and Mack (2003), consists of fossiliferous limestone, cherty limestone, shale, dolomite, and conglomerate deposited in shallow ocean water, with sea level dropping during early Permian time (~290 to 300 million years ago). The units thicken toward the east, into the Orogrande Basin. Conglomerates with clasts of limestone, dolomite, and chert in a sandy, fossiliferous matrix in the upper Bar B Formation in the southern Caballo Mountains indicate a local high point nearby. As the sea retreated, fluvial sandstone, mudstone, siltstone, and conglomerate was deposited by rivers flowing south a broad alluvial plain. The retreat of the sea was oscillatory, so that the contact between the Magdalena Group and the Abo Formation is gradational, with limestone interfingering with Abo redbeds. Floodplain mudstone and siltstone, sinuous channel sandstones, and rare carbonate lake deposits characterize the Permian Abo Formation. Fine-grained windblown sands appear to be more common in the upper part of the section, heralding the overall drying of the climate in New Mexico during Late Permian time.
The Abo Formation grades up into the orange windblown sheet sandstone of the Mesa Blanca Member of the Permian Yeso Formation . The orange sandstone is overlain by a package of dolomite, limestone, red siltstone, and gypsum interpreted to have been deposited in a hypersaline lagoon. The lagoonal deposits grade up into a fossiliferous limestone, indicating a rise in sea level. The uppermost member assigned to the Yeso Formation in the Caballo Mountains is sandstone interbedded with limestone, suggesting a return to lagoonal conditions and a drop in sea level.
Mesozoic
Triassic, Jurassic, and Early to Middle Cretaceous rocks are not preserved in the Caballo Mountains because the area was on the northeastern flank of a Jurassic to middle Cretaceous rift basin (Bisbee Basin/Chihuahua trough). The upper Cretaceous Dakota Sandstone rests unconformably on the Yeso Formation. The Dakota Sandstone contains sandstone, shale, and conglomerate. The lowest part of the section is composed of sand and shale deposits indicative of rivers flowing toward the east to northeast. The top of the Dakota Sandstone contains bioturbated sandstone deposited on a tidal flat along the ocean shore. The Dakota Sandstone grades up into the gray shales and tan siltstones of Rio Saldo Tongue member of the Mancos Shale, signaling a rise in sea level. Sea level subsequently dropped, depositing bioturbated sandstone, shale, and minor coal along the shoreline. Sea level came up again, leaving the black, shaly deltaic deposits of the D-Cross Tongue of the Mancos Shale. Finally, the sea retreated from the region, and the deltaic Gallup Sandstone and fluvial Crevasse Canyon Formation were deposited. The rivers preserved in the Crevasse Canyon Formation flowed toward the northeast. Huge standing tree trunks can be found in the unit on the east side of the Caballo Mountains. Volcanic rock fragments, including andesitic, dacitic, and felsic clasts, found in the Upper Cretaceous sandstones in the Caballo Mountains may have come from southern Arizona (Seager and Mack, 2003; Cumella, 1983).
The latest Cretaceous McRae Formation is an interesting unit that records sedimentation associated with Late Cretaceous arc volcanism and the earliest phases of Laramide deformation. Fluvial channels indicate flow toward the northeast and east-southeast. Debris flow deposits are also common. The basal part is sandstone, conglomerate, mudstone and siliceous volcanic ash. Fossil leaves and petrified wood are common in this unit; dinosaur fossils, including those of Tyrannosaurus rex and Torosaurus, are more rare. The plant assemblage and paleosols in this lower member indicate subhumid, subtropical conditions (Upchurch and Mack, 1998; Buck and Mack, 1995). Intermediate composition volcanic clasts (dacite, trachydacite, trachyandesite, minor rhyolite and rhyolite tuff) in the conglomerates, some of which are boulder-sized, suggest the presence of a nearby stratovolcano that may have collapsed into the Rio Grande rift west of the Caballo Mountains. The Copper Flat Stock near Hillsboro (Figure 8) might be a remnant of that volcano (Seager and Mack, 2003; McMillan, 2004). The upper McRae Formation is purple mudstone, sandstone, quartzite-clast conglomerate, and pink felsic tuff. Red granite and andesite clasts are also found in the quartzite-clast conglomerate; one of the andesite clasts yielded a 40Ar/39Ar age of 70.82 ± 0.42 Ma. The upper McRae Formation contains fewer plant fossils and pedogenic horizons more characteristic of a dry climate (Buck and Mack, 1995).
Paleocene to Eocene Laramide Deformation
Mountain ranges that formed during northeast-directed compressional Laramide deformation in Eocene time in southern New Mexico trend to the northwest and verge toward the northeast. A northwest to north-northwest-trending Laramide basement-cored uplift is preserved in the Caballo Mountains; this uplift, which is northeast of the more extensive Rio Grande uplift exposed in San Diego Mountain and in the San Andres Mountains (Seager and Mack, 1986), shed sediments northward into a broad Laramide basin preserved as the Love Ranch Formation in the modern Jornada del Muerto Basin. The lower two-thirds of the Love Ranch Formation is composed of conglomeratic alluvial fans deposited in an arid to semi-arid environment. This unit records the unroofing of the Laramide highlands, with andestitic clasts common near the base, Abo siltstone and lower to middle Paleozoic carbonate clasts abundant in the middle part of the section, and Proterozoic clasts appearing near the top of the sequence. The upper third of the Love Ranch Formation was deposited by rivers draining toward the north and northeast.
The Love Ranch Formation grades up into the purplish gray volcaniclastic Palm Park Formation. The basal part of the Palm Park Formation is a poorly-sorted conglomerate with clasts of Paleozoic sedimentary, Proterozoic basement, and intermediate composition porphyritic volcanic rocks in a tuffacous muddy matrix. The middle Palm Park Formation is dominated by mudstone and volcanic breccia, and the upper part of the section is mainly fresh water limestone and travertine. The Palm Park Formation appears to represent remnants of distal volcaniclastic aprons shed off of andesitic volcanoes. The carbonates in the upper part of the unit have been interpreted to be hot spring deposits (Chafetz et al.,1991).
Latest Eocene to Neogene Volcanism and Extension
Latest Eocene time marked the beginning of extension and regional volcanism, with volcanic rocks traveling long distances from source to point of deposition across a landscape much more subdued than we see today. Starting about 36 million years ago and continuing until ~27 million year ago, the rhyolitic tuffs, basalt flows, and volcaniclastic sandstone of the Bell Top Formation, Uvas Basaltic Andesite, and Thurman Formation where deposited in the southern Caballo Mountains. Several of the tuffs in the Bell Top Formation and the volcaniclastic sediments and tephras of the Thurman Formation originated from calderas in the Mogollon-Datil volcanic field to the west and northwest of the mountains (McIntosh et al., 1992). The Uvas Basaltic Andesite originated from a shield volcano complex in the Sierra de las Uvas to the southwest of the Caballo Mountains and from fissures in the Point of Rocks area east of the range.
The modern landscape that we see today began to develop following regional volcanism. Based on the analysis of sedimentary deposits in the southern Caballo Mountains, Doña Ana Mountains, San Diego Mountain, and Sierra de las Uvas (Figure 1), Mack et al. (1994a, b) propose that the southern Rio Grande rift has been affected by three episodes of extension beginning at about 36 Ma. The main phases of faulting include (1) latest Eocene to late Oligocene minor faulting coincident with extensive volcanism, (2) late Oligocene to late Miocene rapid extension with minor volcanism, and (3) latest Miocene to early Pliocene continued faulting and volcanism, with each phase disrupting earlier rift basins, and in some cases, reversing the dip of the early rift half-grabens (Figure 12). The south end of the Caballo Mountains once floored one of the early, broad rift basins (Seager et al., 1984). Mack et al. (1994 a,b) note that early unroofing of the Caballo Mountains rift flank uplift, the fourth mountain building episode in the region, is recorded in the Hayner Ranch Formation (approximately 25 to 16 million years old). The lower portion of this formation contains clasts from the youngest part of the stratigraphic section (Oligocene Uvas Basaltic Andesite and Bell Top Tuff), while the upper part of the formation consists of clasts derived from the older Eocene Palm Park and Love Ranch Formations. The basal part of the overlying Rincon Valley Formation (about 16 to 6 million years old) is similar to the Hayner Ranch Formation in that it includes Uvas, Bell Top, Palm Park, and Love Ranch clasts. In contrast, in the middle to upper beds of the Rincon Formation, clasts of Permian Abo Formation and Pennsylvanian Magdalena Group limestones are abundant. No Proterozoic granite clasts are found in the Rincon Valley Formation, except for those thought to be reworked from the Eocene Love Ranch Formation.
The youngest units adjacent to the Caballo Mountains, the 5 to 0.78 million year old Palomas and Camp Rice Formations, unconformably overlie the Rincon Valley Formation (Mack and Seager, 1990). These units preserve alluvial fans shed off the mountains and Ancestral Rio Grande fluvial deposits. Basalt flows that are 2 to 4 million years old are interbedded with the sediments.
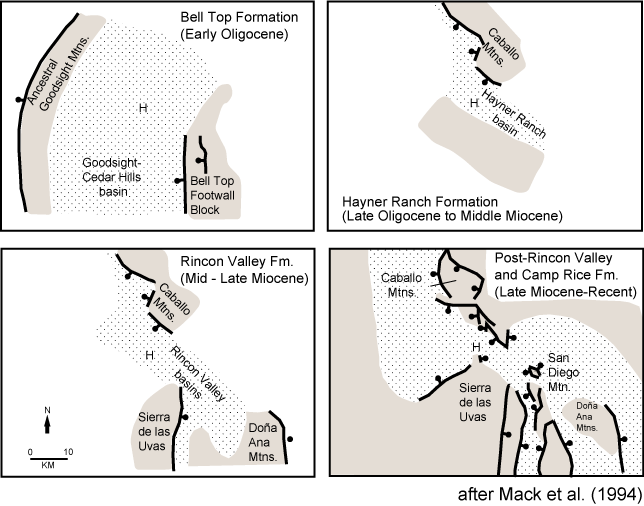
References
- Bauer, P.W., and Lozinsky, R.P., 1986, Proterozoic geology of supracrustal and granitic rocks in the Caballo Mountains, southern New Mexico: new Mexico Geological society Guidebook 37, p. 143-150.
- Bruno, L. and Chafetz H.S.,1988, Depositional environment of the Cable Canyon Sandstone: a mid-Ordovician sand wave complex form southern New Mexico: New Mexico Geological Society Guidebook 39, p. 127-134.
- Buck B.J., and Mack, G.H., 1995, Latest Cretaceous (Maastrictian) aridity indicated by paleosols in the McRae Formation, south central New Mexico: Cretaceous Research, v. 16, p. 559-572
- Chafetz, H.S., Utech, N.M., and Fitzmaurice, S.P., 1991, Differences in the δ18O and δ13C signatures of seasonal laminae comprising travertine stromatolites: Journal of Sedimentary Petrology, v. 61, p. 1015-1028.
- Chapin, C.E. and Seager, W.R., 1975, Evolution of the Rio Grande rift in the Socorro and Las Cruces areas: New mexico Geological Society Guidebook 26, p. 297-321.
- Clemons, R.E., 1984, Nuia siberica Maslov-alias oolite: Geological Society of America Abstracts with Programs, v. 16, p. 473.
- Clemons, R.E., 1991, Petrography and depositional environments of the lower Ordovician El Paso Formation: New Mexico Bureau of Mines and Mineral Resources Bulletin 125, 68 pp.
- Condie, K.C., and Budding, A.J., 1979, Geology and geochemistry of Precambrian rocks, central and south-central New mexico: New Mexico Bureau of Mines and Mineral Resources Memoir 35, 58 pp.
- Cumella, S.P., 1983, Relationship of Upper Cretaceous regressive sandstone units of the San Juan Basin to source area tectonics, in Reynolds, M.W., and Dolly, E.D. (editors), Mesozoic paleogeography of west-central United States: Society of Economic Paleontologists and Mineralogists, Rocky Mountain Section, p. 189-199.
- Karlstrom, K.E., Amato, J.M., Williams, M.L., Heizler, M., Shaw, C., Read, A., and Bauer, P., 2004, Proterozoic tectonic evolution of the New Mexico region: a synthesis, in Mack, G.H. and Giles, K.A., editors, The Geology of New Mexico: A Geologic History: New Mexico Geological Society Special Publication 11, p. 1-34.
- Kelley, V.C., and Silver, C. , 1952, Geology of the Caballo Mountains: University of New Mexico Publications in Geology Number 4, The University of New Mexico Press, Albuqueruqe, New Mexico, 286 pp.
- Kues, B.S., 2001, The Pennsylvanian System in New Mexico--overview with suggestions for revision of stratigraphic nomenclature: New Mexico Geology, v. 23, p. 103-122.
- Kues, B.S., and Giles, K.A., 2004, The late Paleozoic Ancestral Rocky Mountains System in New Mexico, in Mack, G.H. and Giles, K.A., editors, The Geology of New Mexico: A Geologic History: New Mexico Geological Society Special Publication 11, p. 95-136.
- Mack, G.H., 1997, The geology of southern New Mexico: A beginner's guide: University of New Mexico Press, Albuquerque, New Mexico: 176 pp.
- Mack, G.H., and Seager, W.R., 1990, Tectonic control on facies distribution of the Camp Rice and Palomas Formations (Plio-Pleistocene) in the southern Rio Grande rift: Geological Society of America Bulletin, v. 102, p. 45-53.
- Mack, G.H., Nightengale, A.L., Seager, W.R., and Clemons, R.E., 1994a, The Oligocene Goodsight-Cedar Hills half-graben near Las Cruces and its implication to the evolution of the Mogollon-Datil volcanic field and to the southern Rio Grande rift: New Mexico Geological Society, Guidebook 45, p. 135-142
- Mack, G.H., Seager, W.R., and Kieling, J., 1994b, Late Oligocene and Miocene faulting and sedimentation, and evolution of the southern Rio Grande rift, New Mexico: Sedimentary Geology, v. 92, p. 79-96.
- McIntosh, W.C., Chapin, C.E., Ratte, J.C., Sutter, J.F., 1992b, Time-stratigraphic framework of the Eocene-Oligocene Mogollon-Datil volcanic field, southwestern New Mexico: Geological Society of America Bulletin, v. 104, p. 851-871.
- McLemore, V. T., 1986, Geology, geochemistry, and mineralization of syenites in the Red Hills, southern Caballo Mountains, Sierra County, New Mexico—preliminary observations: New Mexico Geological Society, Guidebook 37, p. 151–159.
- McMillan, N.J., 2004, Magmatic record of Laramide subduction and the transition to Tertiary extension: Upper Cretaceous through Eocene igneous rocks in New Mexico: in Mack, G.H. and Giles, K.A., editors, The Geology of New Mexico: A Geologic History: New Mexico Geological Society Special Publication 11, p. 249-270.
- McMillan, N.J., and McLemore, V.T., 2004, Cambrian-Ordovician magmatism and extension in New Mexico and Colorado: New Mexico Bureau of Geology and Mineral Resources Bulletin 160, p. 1-11.
- Mueller, J.E., 1986, History of Elephant Butte Dam and Reservior: New Mexico Geological Society, Guidebook 37, p. 58-59.
- Pope, M.C, 2002, Cherty facies in the Late Ordovician Montoya Group, southern New Mexico and western Texas--implications for Laurentia oceanography and duration of Gondwana glaciation: New Mexico Geological Society Guidebook 53, p. 159-165.
- Seager, W.R., 1986, Third-day road log from Truth or Consequences to southeastern Caballo Mountains and San Diego Mountain via I-25 and the Jornada del Muerto: New Mexico Geological Society, Guidebook 37, p. 35-52.
- Seager, W.R., and Mack, G.H., 2003, Geology of the Caballo Mountains, New Mexico: New Mexico Bureau of Geology and Mineral Resources Memoir 49, 135 pp.
- Seager, W.R., and Mack, G.H., 1986, Laramide paleotectonics of southern New Mexico, in Peterson, J.A., ed., Paleotectonics and Sedimentation in the Rocky Mountain Region: American Association of Petroleum Geologists, Memoir 41, p. 669-685.
- Seager, W.R., Clemons, R.E., Hawley, J.W., and Kelley, R.E., 1982, Geology of the northwest part of the Las Cruces 1°x 2° sheet, New Mexico: New Mexico Bureau of Mines and Mineral Resources Geologic Map 53, scale 1:125,000.
- Seager, W.R., Mack, G.H., Raimonde, M.S., and Ryan, R.G., 1986, Laramide basement-cored uplift and basins in south-central New Mexico: New Mexico Geological Society, Guidebook 37, p. 123-130.
- Seager, W.R., Shafiqullah, M., Hawley, J.W., and Marvin, R.F., 1984, New K-Ar dates from basalts and the evolution of the southern Rio Grande rift: Geological Society of America Bulletin, v. 95, p. 87-99.
- Upchurch, G.R., Jr., and Mack, G.H., 1998, Latest Cretaceous leaf megafloras from the Jose Creek Member, McRae Formation of New Mexico: New Mexico Geological Society Guidebook 49, p. 209-222.
- Wilks, M.E., compiler, 2005, New Mexico Geologic Highway Map: New Mexico Geological Society and New Mexico Bureau of Geology and Mineral Resources, scale1:1,000,000.