
Living Desert Zoo and Gardens State Park
modified from McLemore, V.T., 2001, Living Desert Zoo and Gardens State Park: New Mexico Geology, v. 23, p.42-50.
Introduction
The Living Desert Zoo and Gardens State Park was established in 1971 to preserve and display the wide variety of animals, plants, and natural environments found in the Chihuahuan Desert. Located north of Carlsbad on US–285 (Fig. 1), Living Desert Zoo and Gardens State Park offers a variety of outdoor and indoor exhibits depicting the natural, geologic, archaeological, historical, and mineral wealth of the area.
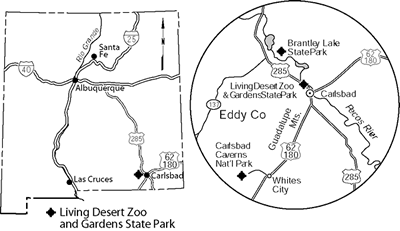
The Chihuahuan Desert covers nearly 200,000 mi2 of northern Mexico, west Texas, and southern New Mexico and is the second largest desert in North America (Fig. 2). It is also the highest desert in North America, with elevations ranging from 1,970 to 5,500 ft. Most of the area receives less than 10 inches of rainfall per year, which comes primarily as monsoons in the summer months, although higher elevations may receive as much as 20 inches per year (McClurg and McClurg, 1990). The first stop on this tour through the Chihuahuan Desert is the Visitor Center, which houses the museum gift shop, information booth, restrooms and lounge, picnic tables, mineral displays, and other educational exhibits on the geology, botany, and wildlife of deserts.
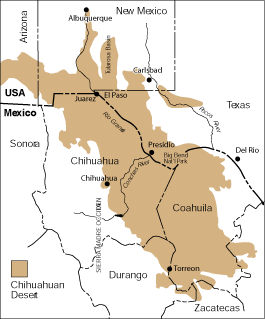
From the Visitor Center a 1.3-mi, self-guided, paved trail passes through reconstructions of a variety of ecosystems present in the Chihuahuan Desert (Fig. 2), including sand hills, an aviary, desert uplands, arroyo, and piñon-juniper forest (McClurg and McClurg, 1990). Each area on the trail has interpretative signs describing the natural environment and wildlife that commonly inhabit it; plants native to the ecosystem are identified by markers. The tour begins with the Sand Hills exhibit, depicting an area east of Carlsbad that consists of Quaternary sand dunes. The Aviary includes a walk-through exhibit of common birds. Several species of native cats (bobcat, mountain lion, and cougar), owls (barn and burrowing), eagles (golden and bald), and hawks (Harris, Swainson’s, and redtail) are housed in specially designed habitat areas near the Aviary. From the Aviary, the trail passes through the Gypsum Hills and Desert Uplands exhibits, made up of local selenite (clear, crystalline gypsum), limestone, and sand, and then through the Desert Arroyo exhibit. Dry streambeds, called arroyos, are common throughout the desert and may quickly funnel rainfall, resulting in flash floods. Javelinas (collared peccary) live in an enclosure near the Desert Arroyo exhibit, as they commonly live in shallow caves and pockets formed by floodwaters in desert arroyos. The mountainous Piñon–Juniper Forest exhibit follows where live bears are housed in a special enclosure. Look carefully because they don’t always play for the spectators but hide in their caves. The trail then goes through the Nocturnal Animals exhibit (salamander, bats, ringtail cat, and kangaroo rat), the popular Mexican Wolf Yard and the Big Game Yards (elk, pronghorn, bison, and mule and white deer). An overlook offers excellent views of Carlsbad and the Pecos River valley. The Prairie Dog Village of fat, barking prairie dogs and the Waterfowl Pond also are popular attractions. The last exhibit on the tour loop is the Reptile House, which houses several varieties of snakes and lizards, including a Gila monster. The trail passes by the Mescal Pit, a rock-rimmed pit or outdoor oven used by Mescalero Apache Indians to cook agave or mescal (McClurg and McClurg, 1990). Each May the Mescalero Apache Indians recreate their historic mescal roasting ceremony, and after four days of cooking they offer the sweet mescal to visitors to taste. The indoor cactus and succulent greenhouse adjacent to the Visitor Center includes the Succulents of the World exhibit and houses different varieties of plants, including cacti from the Southwest as well as from other desert environments in the world.
The park accepts only wild animals that have been injured or are otherwise unable to survive in the wild on their own, and the animals must be native to the Southwest. Many of these animals have had gunshot wounds , have lost toes because of traps, were hit by cars, or were injured by fire. As such, the park's animal population varies from month to month depending on releases, births, deaths, and transfers (Taylor, 1986).
Geology
The various exhibits throughout the park demonstrate the importance of geologic processes in forming the landscape that the “living desert” depends upon. Many of the landforms formed over millions of years.
Proterozoic granite and metamorphic rocks lie at a depth of approximately 11,200 ft and are overlain by approximately 2,700 ft of mostly limestone and dolomite of Cambrian to Upper Permian age (about 550–248 m.y.; Hayes, 1964). Most of the Cambrian–Permian rocks were deposited on wide, shallow-marine shelves (Hayes, 1964; Adams, 1965).
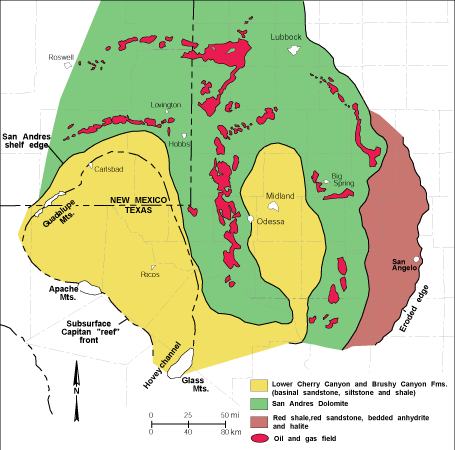
In Pennsylvanian–Permian time (325–245 m.y.) North America, including southeastern New Mexico, was at equatorial latitudes and was covered by a shallow tropical ocean. Deep marine basins were formed south and west of Carlsbad as seas encroached upon shallow-marine shelves depositing the Abo, Hueco, and Yeso Formations. The Delaware Basin (Foster, 1983; Hill, 1996; Fig. 3) formed during the Pennsylvanian Ouachita–Marathon orogeny (a mountain-building event) as part of the larger Permian Basin and was filled by 1,600–2,200 ft of limestones, siltstones, sandstones, and interbedded dark shale. During much of the Permian, the Delaware Basin was a normal marine basin; however, during late Guadalupian time the basin became restricted. The basin consists of three parts: the basin, basin margin, and shelf (Hayes, 1964; Fig. 4), which changed geographic position with time as the basin subsided, filled, and dried out. During the earlier Leonardian Epoch, alternating beds of gypsum, sandstone, and dolomitic limestone of the Yeso Formation were deposited along the shorelines (basin margin), and the thin-bedded black limestones of the Bone Spring Limestone were deposited deeper in the basin (Fig. 4). Later, during the Guadalupian Epoch, the Delaware Basin continued subsiding slowly, the shoreline moved north, and small reefs began to form the Goat Seep Dolomite as the Brushy Canyon and Cherry Canyon Formations were deposited in the basin (Fig. 4). Many of the region's important oil and gas fields, including some near Carlsbad, produce from these rocks.
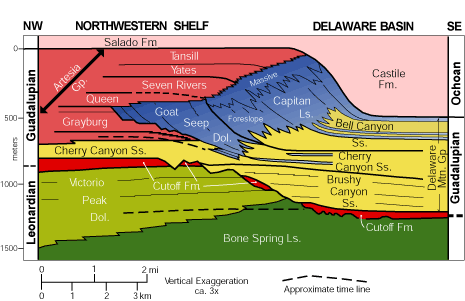
Seas continued to cover much of southeastern New Mexico and west Texas depositing more limestone, dolomite, and gypsum-anhydrite (the Artesia Group; Fig. 4). As the basin subsided rapidly, barrier reefs formed as three distinct facies developed. The lagoon and back reef facies consisted of limestones and dolomites belonging to the Queen and Grayburg Formations. The reef facies with algal and sponge skeletons formed the Goat Seep Dolomite. The offshore basin facies consisted of quartz sandstones of the Cherry Canyon Formation. The largest reef, the Capitan Limestone was deposited along the rim of the basin and rapidly grew toward the basin center (Fig. 4). The Carlsbad Group was deposited along the edges of the basin, and the Bell Canyon Formation was deposited offshore.
The large limestone reef, the Capitan Limestone, formed in the warm tropical seas south of the Carlsbad area at the margin between the shelves and basins (Fig. 4, 5). Present-day limestone reefs are built by various organisms, such as corals and algae, that secrete limy skeletons that are anchored to the sea bottom. As older organisms die, others build their skeletons on top, eventually forming the reef that acts as a barrier to the sea. The Permian (Capitan Limestone) reef is unique because it is composed predominantly of sponges and algae. In addition, at McKittrick Canyon, the entire cross section through the reef (back reef to basin facies) is exposed, making it one of the few places in the world where an exhumed reef can be seen. The Capitan Limestone reef is one of the most famous reefs in the world and is exposed at the surface along the escarpment between Carlsbad Caverns National Park and Guadalupe Peak, southwest of Living Desert Zoo and Gardens State Park. From Carlsbad, the reef trend continues east-northeast in the subsurface. The deep marine Delaware Basin lies south of the Capitan reef(Fig. 3).
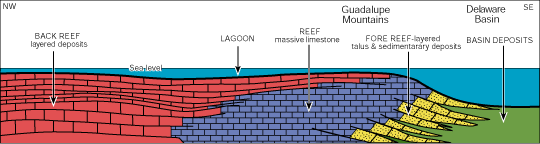
During Late Permian time, continued sedimentation and a drop in sea level allowed for the development of sabkha environments (deposition above normal high tides in an arid environment), the drying of the basin, and the formation of an extensive evaporite sequence (Castile and Salado Formations; Fig. 4) on top of older Permian sediments. When a volume of seawater is evaporated, about 75% of the water is removed by evaporation before anhydrite precipitates and nearly 90% of the water must evaporate before the salts will precipitate. More than 4,000 ft of salt, potash, and anhydrite were deposited during this time (Salado Formation), and these rocks contain economically important salt and potash resources (Barker and Austin, 1999). The last records of the Permian sea are found in the Rustler and Dewey Lake Formations, which consist of marginal marine and continental deposits.
Living Desert Zoo and Gardens State Park is on top of a ridge of hills known as the Ocotillo Hills, northwest of and overlooking Carlsbad. The hills are anticlines that may be draped over earlier reef mounds. The Ocotillo Hills consist of Permian Tansill and Yates Formations. The Tansill Formation consists predominantly of dolomites overlain by gray siltstones that were deposited in shallow-marine-shelf and coastal-plain environments about 260 m.y. ago. The Yates Formation consists of rusty yellow sandstone, siltstone, dolomite, limestone, and evaporites and is exposed mostly in the arroyos below the state park (Motts, 1962). The contact between the Tansill and Yates Formations is exposed in a road cut along the access road to the state park (Anonymous, 1982). Some of the region's important oil and gas fields occur in these rocks.
Petroleum is generated from source rocks rich in organic material and then migrates into reservoir rocks where it is trapped. Permian units are good source rocks because they were deposited when New Mexico was a tropical environment; thus the rocks are rich in organic material. In southeastern New Mexico, oil and gas are produced from many reservoirs of Cambrian through Permian age and from many rock types ranging from sandstone to limestone to dolomite. Petroleum is less dense than water in the pore spaces of the reservoir rocks and tends to migrate updip until it is trapped by an impermeable shale, sandstone, limestone, evaporites, or sealing faults (Broadhead, 1987, 1993). Petroleum also can accumulate at the top of anticlines or in stratigraphic traps, where one type of deposit changes to another (Broadhead, 1987, 1993). These structural and stratigraphic traps are common in the Carlsbad area.
The Avalon (Delaware) oil field is east of Carlsbad and first produced in 1977 (Cantrell and Kane, 1993). The field produces from the upper Cherry Canyon Formation at a 2,600-ft depth and from the lower Cherry Canyon/upper Brushy Canyon Formations at a 3,400-ft depth. The upper Cherry Canyon consists of subarkosic sandstone, whereas the lower Cherry Canyon/upper Brushy Canyon consists of subarkosic siltstones.
Triassic continental fluvial and lacustrine sediments were deposited on top of the Permian rocks. Marine seas returned to New Mexico during Cretaceous time, but only scattered remnants of these deposits are found in the subsurface of southeastern New Mexico (Kues and Lucas, 1993). Most of these rocks have been removed by erosion in the last several million years.
The middle Miocene to early Pliocene Ogallala Formation, about 4–12 m.y. old, overlies the remaining Mesozoic sedimentary rocks and is one of the most widespread units in the United States, extending from South Dakota to southern New Mexico and northwestern Texas. It is the prime reservoir of ground water throughout the region and is responsible for man changing the appearance and character of the Great Plains. The Ogallala Formation is as much as 400 ft thick in southeastern New Mexico and consists of fluvial sandy clay, siltstone, sandstone, and local gravel valley-filling deposits interbedded with eolian sand and silt and playa-lake deposits. These rocks were derived from a continuous chain of mountains extending from the Rocky Mountains in Colorado and northern New Mexico to Trans-Pecos Texas (Reeves, 1972; Hawley, 1984, 1993a,b). Caliche beds are common throughout the unit. Caliche is composed of fractured and permeable deposits of gravel, sand, silt, and clay that are cemented by calcium carbonate (McGrath and Hawley, 1987).
Geomorphic development
Geologic, or more specifically geomorphic, processes formed complex landforms and other topographic features in the Carlsbad area (Hawley, 1993a) that are illustrated in the various exhibits at the state park. There are four major geomorphic processes: regional uplift, erosion, solution-subsidence (including cave formation), and formation of sand dunes.
One major geomorphic process in the Carlsbad area is the formation of the Guadalupe, Sacramento, and Delaware Mountains to the south and west. Regional uplift of the Carlsbad area occurred during the Laramide tectonic event in the early Tertiary (Erdlac, 1993). This was followed by continued uplift, tilting, and faulting during Basin and Range extension. The several thousand feet of topographic relief between the mountains and plains seen today had developed by 12–13 m.y. ago (Hawley, 1993a). The changes in vegetation, wildlife, and geology between the lower elevations of the desert and the piñon-juniper forest of the higher mountains are described in the state park's Desert Uplands and Piñon–Juniper Forest exhibits. The upper elevations tend to have slightly lower temperatures and more moisture, which is ideal for growth of piñon pine, juniper trees, and other high desert and mountain plants. Deep steep-sided canyons provide a diversity of wildlife and vegetation. Ponderosa pine, Douglas-fir, and aspen grow at the higher elevations in the Guadalupe, Sacramento, and Delaware Mountains.
Significant erosion and mass wasting along streams and rivers are the second most important geomorphic process in the Carlsbad area (Hawley, 1993a), and they are illustrated by the Desert Arroyo exhibit at the state park. Arroyos are dry creek beds cut into the landscape by water during heavy rainfall. During thunderstorms, arroyos quickly fill with flowing water, often as flash floods, that erodes into the landscape and moves material downstream. Conglomerates and mudstones typically form the banks of the arroyos. The Desert Arroyo exhibit at the state park illustrates the dependency of wildlife on the thick vegetation that grows in arroyos and provides food, shade, and cover. The arroyos in the Carlsbad area provide geologists with clues as to the formation of the lower Pecos River (Hawley, 1993a). The Pecos River is responsible for long-term removal of sediments and solutes over time and has effectively lowered the landscape. The river probably developed during the Pliocene time (5.3–1.8 m.y.; McLemore, in press).
Solution-subsidence is the third most important geomorphic process in the Carlsbad area (Hawley, 1993a) and is illustrated by the Gypsum Hills and Desert Uplands exhibits. Dissolution of the highly soluble limestone, gypsum, and other evaporites creates natural holes or caves or even larger caverns in the rock, as seen in the exhibit. These underground cavities cause unstable surfaces and create sinkholes. This results in karst topography. Two periods of major dissolution and subsidence occurred in the Carlsbad area since Late Permian time, the first in the Triassic–Jurassic (245–146 m.y.) and the second in the Tertiary–Quaternary (65 m.y.–present; Bachman, 1984). The spectacular landforms in the Carlsbad area, the Guadalupe Ridge and the Capitan Reef escarpment, are a result of removal of overlying sedimentary rocks by dissolution and erosion (Hawley, 1993a). Two processes may be responsible for dissolution and subsidence in the Carlsbad area. Rainwater percolates through crevices in the surface (joints, bedding planes, fractures) and begins to dissolve limestone, gypsum, and other evaporites. Surface water in streams can widen these crevices. Rainwater in contact with CO2 from the atmosphere can form a weak carbonic acid, which further dissolves the rocks. A second process is dissolution by sulfuric acid. Sulfuric acid may have been formed by the oxidation of hydrogen sulfide that migrated upward from deep in the basin along faults (Hill, 1987; Crawford, 1993).
The selenite (crystalline gypsum) slabs used to reconstruct part of the Gypsum Hills along the trail came from the Yeso Hills south of Carlsbad, where planar masses of selenite form beds in part of the Castile Formation. Selenite crystals as much as 18 ft long and 6 ft wide are reported (Crawford, 1993). Early settlers used selenite as windowpanes, and selenite was once quarried for the manufacture of capacitors during World War II (Crawford, 1993). The origin of these large selenite crystals is uncertain. The selenite may have formed by local hydration of anhydrite along faults where basin fluids migrated upward and encountered the Castile evaporites. Another possible formation of the selenite is by dissolution of anhydrite by sulfuric acid, followed by precipitation of selenite. The striations along the selenite crystals are due to modern weathering.
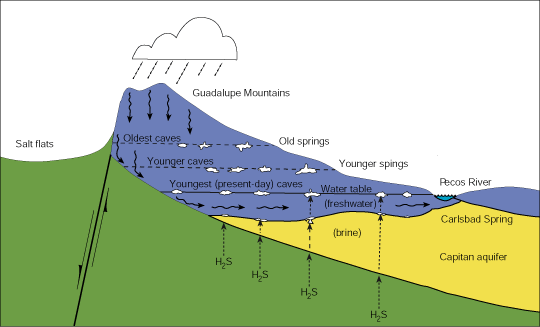
Solution-subsidence in the Carlsbad area also resulted in the formation of extensive caves and cavern systems in the Capitan Limestone; Carlsbad Caverns are the prime example. Processes similar that created the dissolution features (i.e., surface-water dissolution and/or dissolution by sulfuric acid) created the caves and caverns (Hill, 1987). 40Ar/39Ar dating of alunite that formed during the early development of the caves indicates that the Big Room of Carlsbad Caverns formed 4.0–3.9 m.y. ago, whereas Lechuguilla Cave formed 6.0–5.7 m.y. ago and three other caves in the area formed 11.3–6.0 m.y. ago (Polyak et al., 1998). These ages suggest that the water table dropped from 11.3 m.y. ago to the present, possibly related to tectonic uplift and tilting of the Guadalupe Mountains. Some of the caves and caverns are actively forming today. Surface-water dissolution most likely started cave formation in the Guadalupe Mountains, perhaps as early as the Permian (Jagnow and Jagnow, 1992). Extensive cavern formation occurred when the Guadalupe Mountains were uplifted, about 6 m.y. ago. The abundance of gypsum in the caverns supports an origin by sulfuric acid dissolution (Hill, 1987; Jagnow and Jagnow, 1992). Brines rich in hydrogen sulfide, possibly as a byproduct of microbes recycling hydrocarbons (Hill, 1996), seeped upward from the deeper basin along fractures, faults, bedding planes, and other permeable pathways. Hydrogen sulfide combines with the fresh ground water and air to form sulfuric acid, which quickly dissolves the enclosing limestone. As the fresh ground-water table dropped—due to a drier climate, erosion by the Pecos River, and regional uplift—new levels of caverns formed (Fig. 6).
A fourth geomorphic process also contributed to the landscape in the Carlsbad area and is seen in the Sand Hills exhibit. Sand dunes, or moving masses of windblown sand and silt, commonly overlie the Ogallala Formation and are common in most deserts. Sand dunes form in a region that is generally devoid of vegetation and has an abundance of loose sand and topographic features that allow the sand particles to lose their momentum and settle. Any number of objects, such as shrubs, rocks, or fence posts, can obstruct the wind force, causing sand to pile up in drifts and ultimately large dunes. The composition of the sand depends upon the rock types found in the area. The wind picks up pieces of sand and dirt eroded from the surface and hurls the particles against rock exposures, breaking off more particles. Some of these sand dunes can be quite thick, from a few inches to over 60 ft. Frequent wind shifts may uncover or cover plant roots and animal burrows. In wet periods, vegetation will grow on the dunes and somewhat stabilize them. The wind moves the sand, forming new dunes almost daily. Geologists can tell from the shape of the dunes which way the wind is blowing. The windward face of a dune has a gradual slope. Sand blown from the windward face is deposited on the steeply sloping leeward side. Sand on the leeward side or slip face is subject to constant change, avalanching when the sand reaches its maximum angle of repose, at 34°. Impossible as it may seem, no slip face can exceed a 34° angle. Most of the dunes in southeastern New Mexico are crescent shaped in plan view because they formed by constant wind predominantly from one direction across a flat desert floor; these are called barchan dunes. Barchan dunes are common at Oasis State Park near Portales (McLemore, 1998). Parabolic dunes, which also are crescent shaped, are locally formed by wind blowing from the opposite direction. Head and tail dunes grow in the protected air pockets near rocks or shrubs. Longitudinal dunes form long, linear ridges of sand by a strong, persistent prevailing wind.
References
- Adams, J. E., 1965, Stratigraphic-tectonic development of Delaware Basin: American Association of Petroleum Geologists, Bulletin, v. 49, pp. 2140–2148.
- Anonymous, 1982, Living Desert State Park, Zoological–Botanical Gardens: New Mexico Geology, v. 4, pp. 25–26.
- Bachman, G. O., 1984, Regional geology of Ochoan evaporites, northern part of Delaware Basin: New Mexico Bureau of Mines and Mineral Resources, Circular 184, 22 pp.
- Barker, J. M., and Austin, G. S., 1999, Overview of the Carlsbad potash district; in Potash resources at WIPP site, New Mexico: New Mexico Bureau of Mines and Mineral Resources, Circular 207, pp. 7–16.
- Broadhead, R. F., 1987, Petroleum exploration targets in New Mexico for the late 1980s and beyond: New Mexico Geology, v. 9, pp. 31–36.
- Broadhead, R. F., 1993, Have you ever wondered why New Mexico is so rich in oil and gas?: Lite Geology, Winter, 3 pp.
- Cantrell, D. L., and Kane, T. V., 1993, Production history and geology of the Avalon (Delaware) field; in Love, D. W., Hawley, J. W., Kues, B. S., Adams, J. W., Austin, G. S., and Barker, J. M. (eds.), Carlsbad region, New Mexico and west Texas: New Mexico Geological Society, Guidebook 44, pp. 81–82.
- Christiansen, P. W., 1989, The story of oil in New Mexico: New Mexico Bureau of Mines and Mineral Resources, Scenic Trips to the Geologic Past, no. 14, 112 pp.
- Crawford, J. E., 1993, K Hill and Yeso Hills selenite occurrence; in Love, D. W., Hawley, J. W., Kues, B. S., Adams, J. W., Austin, G. S., and Barker, J. M. (eds.), Carlsbad region, New Mexico and west Texas: New Mexico Geological Society, Guidebook 44, pp. 8–10.
- DeFord, R. K., and Wahlstrom, E. A., 1932, Hobbs field, Lea County, New Mexico: American Association of Petroleum Geologists, Bulletin, v. 16, pp. 51–90.
- Energy Information Administration, 2000, U.S. crude oil, natural gas, and natural gas liquids reserves 1999 annual report: U.S. Department of Energy, Report DOE/EIA-0216(99), pp. 20, 31.
- Erdlac, R. J., Jr., 1993, Small-scale structures in the Guadalupe Mountains region—Implications for Laramide stress trends in the Permian Basin; in Love, D. W., Hawley, J. W., Kues, B. S., Adams, J. W., Austin, G. S., and Barker, J. M. (eds.), Carlsbad region, New Mexico and west Texas: New Mexico Geological Society, Guidebook 44, pp. 167–174.
- Foster, A. B., 1983, Guadalupe Mountains National Park; in Harris, A., and Tuttle, E. (eds.), Geology of National Parks: Kendall/Hunt Publishing Co., Dubuque, IA, pp. 409–417.
- Hawley, J. W., 1984, The Ogallala Formation in eastern New Mexico—proceedings of the Ogallala aquifer symposium II: Texas Tech University, Water Resources Center, pp. 157–176.
- Hawley, J. W., 1993a, Overview of the geomorphic history of the Carlsbad area; in Love, D. W., Hawley, J. W., Kues, B. S., Adams, J. W., Austin, G. S., and Barker, J. M. (eds.), Carlsbad region, New Mexico and west Texas: New Mexico Geological Society, Guidebook 44, pp. 2–3.
- Hawley, J. W., 1993b, The Ogallala and Gatuña Formations in the southeastern New Mexico region, a progress report; in Love, D. W., Hawley, J. W., Kues, B. S., Adams, J. W., Austin, G. S., and Barker, J. M. (eds.), Carlsbad region, New Mexico and west Texas: New Mexico Geological Society, Guidebook 44, pp. 261–269.
- Hayes, P. T., 1964, Geology of the Guadalupe Mountains, New Mexico: U.S. Geological Survey, Professional Paper 446, 65 pp.
- Hill, C. A., 1987, Geology of Carlsbad Cavern and other caves in the Guadalupe Mountains, New Mexico and Texas: New Mexico Bureau of Mines and Mineral Resources, Bulletin 117, 150 pp.
- Hill, C. A., 1996, Geology of the Delaware Basin, Guadalupe, Apache, and Glass Mountains, New Mexico and west Texas: Permian Basin Section–SEPM, Publication no. 96–39, 480 pp.
- Jagnow, D. H., and Jagnow, R. R., 1992, Stories from stones—the geology of the Guadalupe Mountains: Carlsbad Caverns–Guadalupe Mountains Association, Carlsbad, 41 pp.
- Julyan, R., 1996, The place names of New Mexico: University of New Mexico Press, Albuquerque, 385 pp.
- King, P. B., 1948, Geology of the southern Guadalupe Mountains, Texas: U.S. Geological Survey, Professional Paper 215, 183 pp.
- Kues, B. S., and Lucas, S. G., 1993, Stratigraphy, paleontology and correlation of Lower Cretaceous exposures in southeastern New Mexico; in Love, D. W., Hawley, J. W., Kues, B. S., Adams, J. W., Austin, G. S., and Barker, J. M.( eds.), Carlsbad region, New Mexico and west Texas: New Mexico Geological Society, Guidebook 44, pp. 245–260.
- McClurg, D., and McClurg, J, 1990, Living Desert State Park Visitor’s Guide: Living Desert State Park, Carlsbad, 28 pp.
- McGrath, D. B., and Hawley, J. W., 1987, Geomorphic evolution and soil-geomorphic relationships in the Socorro area, central New Mexico; in McLemore, V. T., and Bowie, M. R., (comps.), Guidebook to the Socorro area, New Mexico: New Mexico Bureau of Mines and Mineral Resources, pp. 55–67.
- McLemore, V. T., 1993, Globe Plaster Mining Co., Oriental, New Mexico; in Love, D. W., Hawley, J. W., Kues, B. S., Adams, J. W., Austin, G. S., and Barker, J. M. (eds.), Carlsbad region, New Mexico and west Texas: New Mexico Geological Society, Guidebook 44, p. 80.
- McLemore, V. T., 1998, Oasis State Park: New Mexico Geology, v. 20, pp. 106–108.
- McLemore, V. T., in press, Brantley Lake State Park: New Mexico Geology.
- McLemore, V. T., and Hoffman, G., in preparation, Mining districts of New Mexico—distribution and production: New Mexico Bureau of Mines and Mineral Resources, Resource Map.
- Motts, W. S., 1962, Geology of the West Carlsbad quadrangle, New Mexico: U.S. Geological Survey, Geologic Quadrangle Map GQ-167, scale 1:62,500.
- Polyak, V. J., McIntosh, W. C., Güven, N., and Provencio, P., 1998, Age and origin of Carlsbad Cavern and related caves from 40Ar/39Ar of alunite: Science, v. 279, pp. 1919–1922.
- Pray, L. C., 1988, Geologic guide for U.S. Highway 62(180) from El Paso to southern Guadalupe Mountains at the junction of US 26–180 with Texas 54 from Van Horn; in Sarg, J. F., Rossen, C., Lehmann, P. J., and Pray, L. C. (eds.), Geologic guide to the western escarpment, Guadalupe Mountains, Texas: Society for Sedimentary Geology (SEPM), Permian Basin Section, Publication 88–30, pp. 9–13.
- Reeves, C. C., Jr., 1972, Tertiary–Quaternary stratigraphy and geomorphology of west Texas and southeastern New Mexico; in Kelley, V. C., and Trauger, F. D. (eds.), East-central New Mexico: New Mexico Geological Society, Guidebook 23, pp. 108–117.
- Schmidt, R. H., Jr., 1979, A climatic delineation of the “real” Chihuahuan Desert: Journal of Arid Environments, v. 2, pp. 243–250.
- Tyrrell, W. W., Jr., 1969, Criteria useful in interpreting environments of unlike but time-equivalent carbonate units (Tansill–Capitan–Lamar), Capitan reef complex, west Texas and New Mexico; in Friedman, G. M. (ed.), Depositional environments in carbonate rocks: SEPM, Special Publication no. 14, pp. 80–97.
- Ward, R. F., Kendall, C. G. St. C., and Harris, P. M., 1986, Upper Permian (Guadalupian) facies and their association with hydrocarbons—Permian Basin, west Texas and New Mexico: American Association of Petroleum Geologists, Bulletin, v. 70, pp. 239–262.