
Kilbourne Hole
Location
Note: Kilbourne Hole is now part of the Desert Peaks - Organ Mountains National Monument and collection of materials is not allowed until a resource assessment is complete.
Kilbourne Hole in south-central New Mexico (Figure 1) is a classic example of a maar crater (Figure 2) that formed as a result of the explosive interaction of hot basaltic magma with groundwater during a volcanic eruption. When the steam-saturated eruption column that forms during an explosive event gravitationally collapses, a ring-shaped surge travels radially outward along the ground away from the vent. The stratified, cross-bedded pyroclastic surge deposits around the crater at Kilbourne Hole are spectacular (Figure 3). The surge deposits may have formed as a consequence of a series of steam explosions during the emplacement of the basalt.
Kilbourne Hole is unique because of the remarkable abundance of both crustal and mantle (peridotite/olivine-bearing) xenoliths that are in basalt bombs ejected during the eruption. Xenoliths are inclusions of pre-existing rock derived from country rocks, in this case, pieces of mantle and crust, that were incorporated into the mafic magma as it moved from a depth of about 40 miles (60 km) to the surface.
As a crow flies, Kilbourne Hole is about 22 miles northwest of El Paso, Texas and is about 25 miles southwest of Las Cruces, New Mexico (Figure 1). The crater can be reached via a series of county dirt roads that can be treacherous when wet. The Bureau of Land Management manages the land at the rim as part of the Desert Peaks - Organ Mountains National Monument, but the floor of the crater is private property.
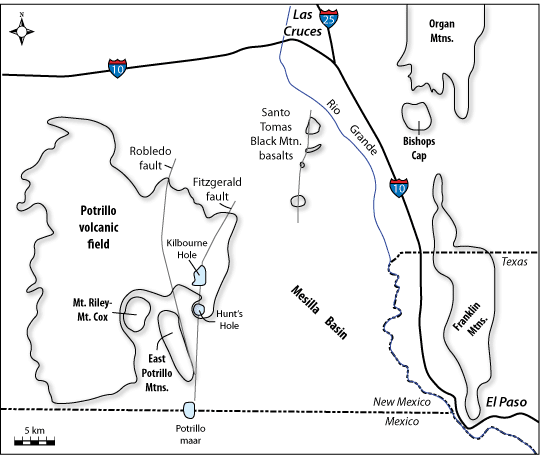
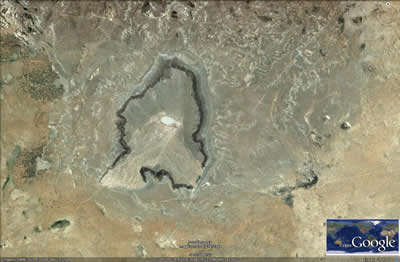
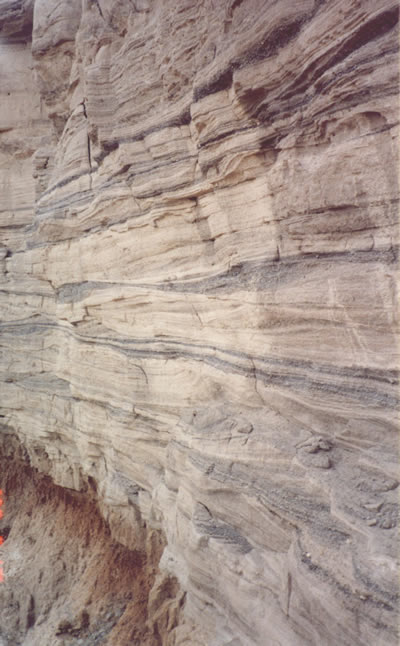
Regional Geologic Setting
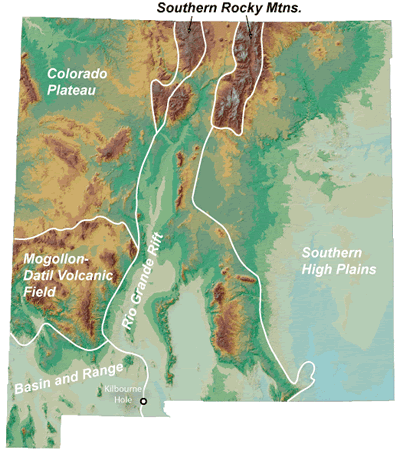
Kilbourne Hole is in the Mesilla Basin, one of a series of linked basins between central Colorado and west Texas that formed starting about 36 million years ago (McMillan, 2004) when the earth’s crust extended or stretched in an east-west direction. Normal faulting associated with extension led to the development of deep asymmetric basins and prominent rift-flank uplifts, producing a continental-scale extensional feature known as the Rio Grande rift (Figure 4). The Franklin and Organ mountains to the east and the East Portillo Mountains to the west of Kilbourne Hole are examples of rift-flank uplifts in south-central New Mexico. The rate of rift flank uplift and basin subsidence likely peaked between 4 and 10 million years ago (Seager and Mack, 1994).
Crustal thinning during extension tends to trigger volcanic eruptions. The basalt lavas at Kilbourne Hole are on the eastern edge of the aerially-extensive Potrillo volcanic field (Figure 1), which was active between 1.2 million and 20,000 years ago (Hawley, 1981; Anthony et al., 1992; Williams, 1999). This youthful volcanism suggests that Rio Grande rift extension is still active in this region. Indeed, the range-bounding normal fault on the east side of the East Potrillo Mountains offsets pyroclastic surge deposits associated with the 180,000-year-old Potrillo maar to the west and south of Kilbourne Hole (Seager and Mack, 1994). The more than 100 vents of the Potrillo volcanic field are aligned along older faults. Kilbourne Hole, Hunt’s Hole, and Potrillo maar are aligned along the Fitzgerald-Robledo fault system (Figure 1; Hoffer, 1976a,b; Parovani and Reid, 1989).
Geologic History
Extension in this part of the Rio Grande rift began about 36 million years ago. Rock debris that eroded from the developing rift-flank highlands, as well as wind-blown and playa lake deposits, accumulated in the subsiding Mesilla Basin. These basin fill deposits, known as the Santa Fe Group, are 1500 to 2000 feet thick beneath Kilbourne Hole (Hawley, 1984; Hawley and Lozinsky, 1993). The uppermost sand, silt, and clay of the Pliocene to early Pleistocene Camp Rice Formation, the youngest unit of the Santa Fe Group in this part of the basin, are exposed in the bottom of Kilbourne Hole. The Camp Rice Formation was deposited by a south-flowing braided river that emptied into a playa lake in the vicinity of El Paso.
The La Mesa surface, a flat surface that developed on top of the Camp Rice Formation, represents the maximum basin fill of the Mesilla Basin at the end of Santa Fe Group deposition about 700,000 years ago (Mack et al., 1994). This surface is about 300 ft above the modern Rio Grande floodplain. The surface formed during a period of landscape stability. Basalt flows from the Portillo volcanic field are intercalated with the upper Camp Rice Formation and lie on the La Mesa surface.
The Rio Grande started to cut down through the older Santa Fe Group deposits after 700,000 years ago in response to both climatic changes and integration of the river system with the Gulf of Mexico. This downcutting was not a continuous process; there were several episodes of downcutting, back-filling, and renewed incision. This episodic development of the river system led to the formation of several terrace levels along the Rio Grande between Las Cruces and El Paso.
Basalt that erupted about 70,000 to 81,000 years ago from a set of vents called the Afton cones located north-northeast of Kilbourne Hole flowed southward. The explosion that formed Kilbourne Hole erupted through the distal edges of the Afton basalt flows, indicating that the crater is younger than 70,000 to 81,000 years old. Pyroclastic surge beds and vent breccia blown from the crater overlie the Afton basalt flow. The crater formed druing the final stages of the eruption (Seager, 1987).
Volcanic Features
Bombs and bomb sags
Volcanic bombs are blobs of molten lava ejected from a volcanic vent. Bombs are at least 2.5 inches in diameter and are often elongated, with spiral surface markings acquired as the bomb cools as it flies though the air (Figure 5).
Bomb sags are common features in the pyroclastic suge beds. The sags form when ejected volcanic bombs impact into the finely stratified surge beds (Figure 6).
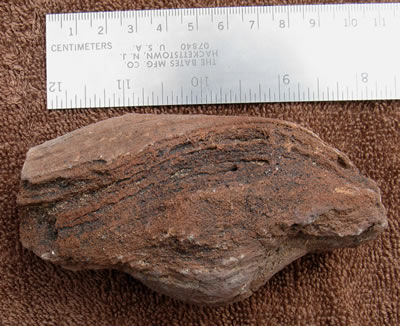
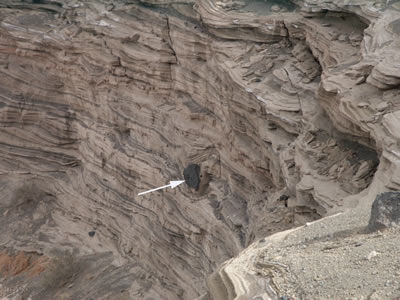
Xenoliths
Many of the volcanic bombs at Kilbourne Hole contain xenoliths. Granulite, charnokite, and anorthosite are common xenoliths in bombs at Kilbourne Hole; these xenoliths are interpreted to represent pieces of the lower to middle crust (Figure 7; Hamblock et al., 2007). The granulite may contain garnet and sillimantite, indicative of a metasedimentary origin, or the granulite may contain pyroxene, suggestive of an igneous origin (Padovani and Reid, 1989; Hamblock et al., 2007). Other upper crustal xenoliths include intermediate and silicic-composition volcanic rocks, clastic sedimentary rocks, basalt and basaltic andesite, and limestone (Padovani and Reid, 1989; French and McMillan, 1996).
Mantle xenoliths (Figure 8) include spinel lherzolite, harzburgite, dunite, and clinopyroxenite. Study of these xenoliths has provided important data on the composition and temperature of the mantle at depths of 40 miles beneath the earth's surface (e.g., Parovani and Reid, 1989; Hamblock et al., 2007). Some olivine in the mantle xenoliths is of sufficient size and clarity to be considered gem-quality peridot, the August birthstone.
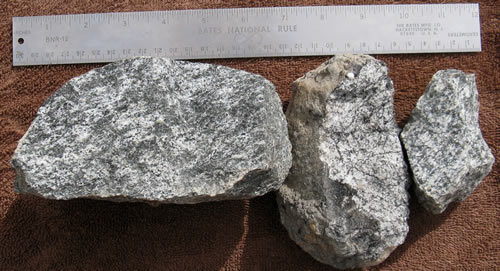
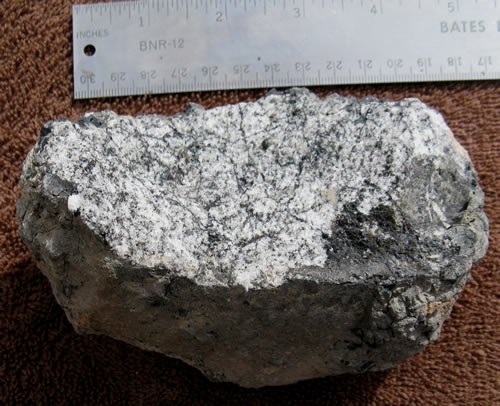
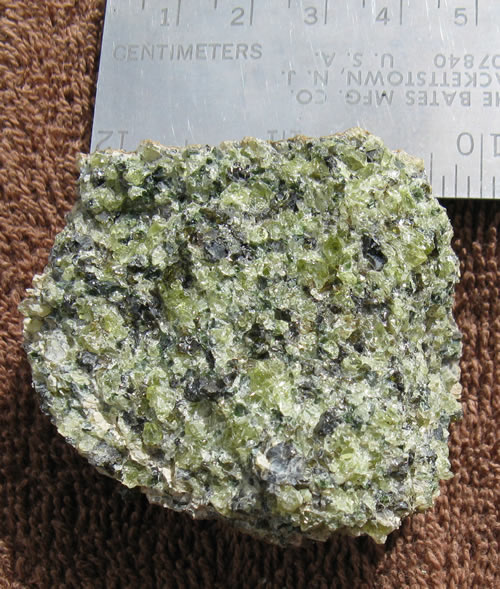
Surge beds
A pyroclastic surge is hot cloud that contains more gas or steam than ash or rock fragments. The turbulent cloud moves close to the ground surface, often leaving a delicately layered and cross-stratified deposit (Figures 3 and 6). The layering forms by unsteady and pulsating turbulence in the cloud.
Hunt’s Hole and Potrillo Maar
Many of the features described above are also present at Hunt’s Hole and Potrillo maar (Figure 9), which are located to the south of Kilbourne Hole. Xenoliths are rare to absent at Hunt’s Hole (Padovani and Reid, 1989), but otherwise the maars are similar. In contrast to Kilbourne Hole, Potrillo maar is not rimmed by a basalt flow, and cinder cones and a younger basalt flow occupy the floor of Potrillo maar (Hoffer, 1976b).
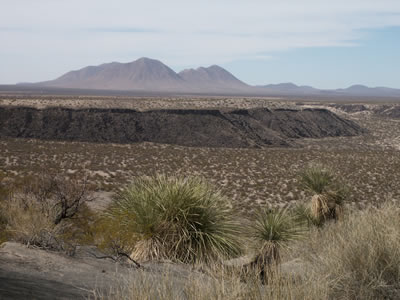
References
- Anthony E. Y., and Poths J., 1992, 3He surface exposure dating and its implications for magma evolution in the Potrillo volcanic field, Rio Grande Rift, New Mexico, USA: Geochimica et Cosmochimica Acta, v. 56, p. 4105-4108.
- French, E.C., IV, and McMillan, N.J., 1996, A lithologic study of xenoliths from the Kilbourne Hole maar, southern New Mexico: New Mexico Geological Society Annual Meeting Proceedings Volume, Socorro, New Mexico, p. 62.
- Hamblock, J. M., Andronicos, C. L., Miller, K. C., Barnes, C. G., Ren, M-H., Averill, M. G., and Anthony, E. Y., 2007, A composite geologic and seismic profile beneath the southern Rio Grande rift, New Mexico, based on xenolith mineralogy, temperature, and pressure: Tectonophysics, v. 442, p. 14-48.
- Hawley, J.C., 1981, Pleistocene and Pliocene history of international boundary area, southern New Mexico, in Hoffer, J.M., and Hoffer, R.L., eds., Geology of the border, southern New Mexico and northern Chihuahua: El Paso Geological Society, p. 26-32.
- Hawley, J. W., 1984, Introduction to hydrogeologic features of the Mesilla Bolson area, Doña Ana County, New Mexico, and El Paso County, Texas: New Mexico Bureau of Geology and Mineral Resources Open-file Report 190, 11 pp.
- Hawley, J. W. and Lozinsky, R. P., 1993, Hydrogeologic framework of the Mesilla Basin in New Mexico and western Texas: New Mexico Bureau of Geology and Mineral Resources Open-file Report 323, 98 pp.
- Hoffer, J.M., 1976a, Geology of the Potrillo basalt field, south-central New Mexico: New Mexico Bureau of Mines and Mineral Resources Circular 149, 30 pp.
- Hoffer, J.M., 1976b, The Potrillo basalt field, south-central New Mexico: New Mexico Geological Society Special Publication 5, p. 89-92.
- Mack, G. H., Salyards, S. L., and James, W. C., 1994, Magnetostratigraphy of the Plio-Pleistocene Camp Rice and Palomas Formations in the Rio Grande rift of southern New Mexico: American Journal of Science: v. 293, pp. 49-77.
- McMillan, N.J., 2004, Magmatic record of Laramide subduction and the transition to Tertiary extension: Upper Cretaceous through Eocene igneous rocks in New Mexico, in, Mack, G.H., and Giles, K.A., eds., The Geology of New Mexico: A Geologic History, New Mexico Geological Society Special Publication 11, p. 249-270.
- Padovani, E.R., and Reid, M.R., 1989, Field guide to Kilbourne Hole maar: New Mexico Bureau of Mines and Mineral Resources Memoir 46, p. 174-185.
- Seager, W.R., 1987, Caldera-like collapse at Kilbourne Hole maar: New Mexico Geology, v. 9, p. 69-73.
- Williams, W.J.W., 1999, Evolution of Quaternary intraplate mafic lavas detailed using 3He surface exposure and 40Ar/39Ar dating, and elemental He, Sr, Nd, and Pb isotopic signature, Potrillo volcanic field, USA and San Quintin volcanic field, Baja California, Mexico, [Ph.D. dissertation], University of Texas at El Paso, 195 pp.