
Brantley Lake State Park
modified from McLemore, V.T., 2001, Brantley Lake State Park: New Mexico Geology, v. 23, no. 4, p. 123-128
Introduction
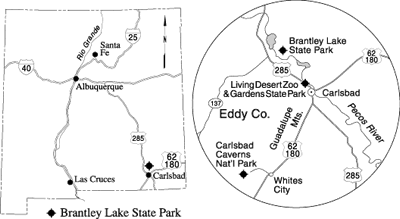
Brantley Lake State Park on the Pecos River was officially opened in November 1989. The park lies 12 mi north of Carlsbad via US–285 (Fig. 1). Brantley Lake is designed to hold 348,540 acre-ft of water. Although the primary functions of the lake are flood control and water storage for irrigation and water commitments to Texas and Mexico, the lake is best known for its water recreation and fishing. The most common fish stocked by the New Mexico Department of Game and Fish include largemouth bass, walleye, channel catfish, trout, sunfish, white bass, bluegill, and crappie. Brantley Wildlife Management Area lies south of the dam, as well as north of Brantley Lake, in the area once occupied by Lake McMillan. The Pecos River valley is a major waterfowl migration route, and many species of birds are present on and near the lake throughout the year.
Geology
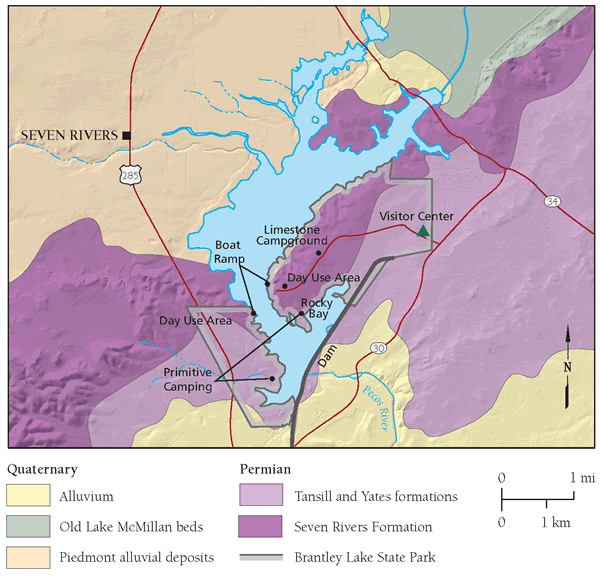
Brantley Lake State Park is similar in geologic setting to Living Desert Zoo and Gardens State Park, located approximately 12 mi to the southeast. Readers are referred to the article on Living Desert Zoo and Gardens State Park printed in New Mexico Geology in May 2001 (McLemore, 2001) for further discussion of the geology and geomorphology of the area.
Quaternary, Tertiary, and Permian sedimentary rocks are exposed at and near Brantley Lake State Park (Fig. 2). Brantley Lake lies on the northwest shelf of the Permian Basin, a structural platform that extends along the basin's northern margin into Texas and slopes into the Delaware Basin (Hayes, 1964; Foster, 1983; Ward et al., 1986). The Delaware Basin (Fig. 3) formed during the Wolfcampian Epoch of the Permian as part of the larger Permian Basin and was filled by 1,600–2,200 ft of limestone, sandstone, evaporite, and interbedded dark shale. The Delaware Basin was mostly enclosed, but marine water did enter the basin. The basin consists of three parts or facies: shelf, basin margin, and basin (Fig. 3; Hayes, 1964), which changed geographic position with time as the basin subsided, filled, and dried out. The rocks change toward the center of the Delaware Basin from a shelf evaporite facies in the northwest to a shelf carbonate facies and reef facies to basin-fill sediments. Where Brantley Lake lies along the Pecos River, the Permian Seven Rivers Formation (Artesia Group) crops out (Kelley, 1971). The eastern shore of the lake consists of local outcrops of limestone, dolostone, mudstone, and gypsum of the Seven Rivers Formation (Fig. 2).

The carbonate and evaporite facies of the Seven Rivers Formation contain many caves and sinkholes, which are not a suitable site for the construction of a dam. The evaporite facies consists of thin, red to reddish-brown gypsum, silt, clay, dolostone, and minor sandstone beds; the red to reddish-brown color is a result of the oxidation of iron oxides. The gypsum was originally deposited as anhydrite, but was altered by circulating ground water (Cox, 1967). Dissolution of the highly soluble gypsum, anhydrite, and other evaporites created natural holes or caves or even larger caverns in the rock. Three caves are located in gypsum units along the bluff east of former Lake McMillan, Coffee Cave, Clarks Caverns, and Homogenized White Cave (Fig. 2). These underground cavities can cause an unstable surface and create sinkholes, similar to those found at Santa Rosa and Bottomless Lakes State Parks (McLemore, 1989, 1999). This results in karst topography. Water leaking from Lake McMillan into local sinkholes threatened to decrease the stored water capacity. Dikes were built along the eastern edge of Lake McMillan in 1908–1909 and 1953–1954 in an attempt to prevent water from leaking into the sinkholes (Cox, 1967).
Two periods of major dissolution and subsidence occurred in the Carlsbad area since the Late Permian, the first in Triassic–Jurassic time and the second in Tertiary–Quaternary time (Bachman, 1984). Two mechanisms may be responsible for this dissolution and subsidence. Rainwater percolating through crevices in the surface (joints, bedding planes, fractures) begins to dissolve limestone, gypsum, and other evaporites. Rivers and streams can widen these crevices. Rainwater coming in contact with carbon dioxide (CO2) found in limestones can form a weak carbonic acid, which further dissolves the rocks. A second mechanism is dissolution by sulfuric acid. Sulfuric acid may have been formed by the oxidation of hydrogen sulfide that migrated from deep in the basin along faults to the surface (Hill, 1987; Crawford, 1993).
Brantley Dam was specifically built where there is a facies change at the surface from permeable evaporite (gypsum, anhydrite, and dolostone) to less permeable dolostone and minor sandstone, siltstone, and shale of the Azotea Tongue of the Seven Rivers Formation (Crouch and Welder, 1988). The carbonate facies, consisting of predominantly gray to grayish-brown dolostone and some local thin pink beds, offers a better site for a dam because the rocks are not as easily dissolved as the evaporites.
Brantley Lake impounds water from the Pecos River, which has its headwaters in the southern Sangre de Cristo Mountains. The Pecos River passes through Villanueva, Santa Rosa Lake, and Sumner Lake State Parks, and it continues southward into west Texas and eventually enters the Rio Grande at Amistad National Recreation Area (Fig. 4). Following the Laramide uplift of the Rocky Mountains, large quantities of eroded material from the mountains were transported by wind and water southeastward and were deposited as large piedmonts or broad, flat to gently sloping surfaces that extended from the mountain front (Hawley, 1993). These piedmonts formed part of what is now known as the Great Plains, and they border the current Pecos River valley on the east. These deposits, known as the Ogallala Formation, were deposited during the Miocene and into the Pliocene. Ogallala Formation deposition ceased during the Pliocene, when the climate became wetter and the source-area drainage was diverted into a series of evolving river valleys. The Pecos River was one of these river systems, and it began cutting into the Ogallala sediments, forming the Pecos River valley, which now separates the Great Plains from the mountains. Only small patches of the Ogallala Formation remain west of the Pecos River.
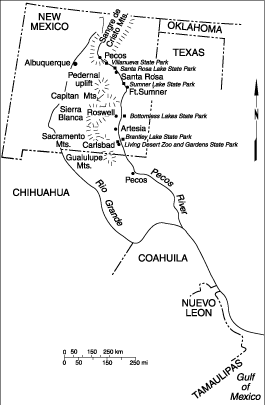
A combination of three geologic processes formed the Pecos River valley: (1) dissolution of underlying rocks, (2) downcutting and lateral erosion due to regional uplift, and (3) changes in climate. The channel of the Pecos River south of Santa Rosa and Roswell (Bottomless Lakes State Park) may have been influenced in part by the dissolution of underlying rocks in a process similar to that which formed Bottomless Lakes and the lakes at Santa Rosa (Harrington, 1957; McLemore, 1989, 1999). Changes in the amount of regional uplift combined with changes in the climate produced the steep-walled, narrow canyons of the Pecos River canyon at Villanueva State Park and northward (Fig. 5; Hawley et al., 1976; Hawley, 1993; McLemore, 1996). Deep, narrow canyons only formed along the upper Pecos River (Fig. 5), where uplift was more pronounced, producing a steeper gradient, and resulting in the river maintaining its course and downcutting into the sedimentary rocks exposed there. In contrast, the Pecos River valley south of Villanueva, including the Brantley Lake area, is broad, because the river has meandered back and forth over time (Fig. 5). Regional uplift was minimal in this area of the river valley, so the gradient is flatter; therefore, the valley remained broad and relatively flat.
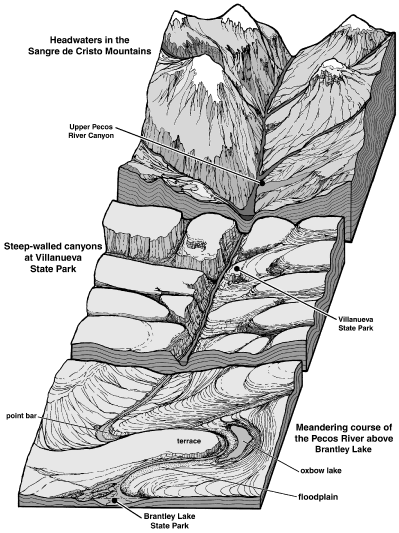
During this period of regional uplift, the climate was affected by episodic changes in precipitation and runoff that were related to cyclic periods of glacial melting (Hawley et al., 1976; Hawley, 1993). When the glaciers melted, greater amounts of water and sediment flowed swiftly downstream from the mountains cutting steep-sided canyons. When glacial melt decreased, local tributary drainage basins supplied most of the water and sediment into the Pecos River, and the river valley began to fill with sediment. Geologists call this process of rivers filling with sediment aggradation.
The present Pecos River channel occupies the lowest point in the valley and is bordered by floodplains, oxbow lakes, and swamps that formed as a result of abandonment of the older Pecos River. Meanders or looplike bends in the river are common along the current floodplain. The meanders widen and flatten the river valley by carving low cliffs called “bluffs” and dumping sediment onto the floodplain (Fig. 5). Erosion is much less along the southern Pecos River than in the north where the gradient is steeper. Much of the northeast side of Brantley Lake consists of surficial river deposits of Quaternary and Tertiary age. These sediments are derived from the Sangre de Cristo, Sacramento, and Capitan Mountains and consist of poorly sorted, poorly consolidated deposits of sand, silt, gravel, and rare cobbles and boulders as much as 35 ft thick. The coarser fragments are well rounded by mechanical abrasion as a result of tumbling in the river over long distances. The river deposited silt, sand, and gravel in channels. Along the inner loop of each meander, the water was shallower and the water velocity was lower than along the outside of the meander; coarser sediments accumulated as point bars. During flood stages, finer-grained material, silt and clay, accumulated as overbank deposits along the floodplain of the river. As the river meandered back and forth and encountered less-erodible material along its course, it abandoned its course and continued to move toward the lowest point in the valley. Cut-off meanders formed oxbow lakes (Fig. 5). The abandoned river deposits formed terraces above the current floodplain. Dams, such as Brantley Dam, and levees were built along the Pecos River, to control floods as well as to store water.
Sand dunes are common throughout and around the state park. They were formed in modern times from wind-blown material. The constant wind moves the sand, forming new dunes almost daily. These sand dunes are reddish brown to tan to cream colored because the sand is composed of medium- to fine-grained, clear quartz and reddish to tan feldspar crystals. Some dunes are white because they consist of white gypsum, clear quartz, and minor feldspar. Locally, these dunes are as much as 60 ft thick (Cox, 1967).
References
- Bachman, G. O., 1984, Regional geology of Ochoan evaporites, northern part of Delaware Basin: New Mexico Bureau of Mines and Mineral Resources, Circular 184, 22 pp.
- Cox, E. R., 1967, Geology and hydrology between Lake McMillan and Carlsbad Springs, Eddy County, New Mexico: U.S. Geological Survey, Water-supply Paper 1828, 48 pp.
- Crawford, J. E., 1993, K Hill and Yeso Hills selenite occurrence; in Love, D. W., Hawley, J. W., Kues, B. S., Adams, J. W., Austin, G. S., and Barker, J. M. (eds.), Carlsbad region, New Mexico and west Texas: New Mexico Geological Society, Guidebook 44, pp. 8–10.
- Crouch, T. M., and Welder, G. E., 1988, Potential hydrologic effects of a drainage system in McMillan delta and water impoundment in Brantley Reservoir, Eddy County, New Mexico: U.S. Geological Survey, Water-resources Investigations, Report 88-4054, 44 pp.
- Foster, A. B., 1983, Guadalupe Mountains National Park; in Harris, A., and Tuttle, E. (eds.), Geology of National Parks: Kendall/Hunt Publishing Co., Dubuque, Iowa, pp. 409–417.
- Harrington, E. R., 1957, Sinkholes, bottomless lakes, and the Pecos River: Scientific Monthly, v. 84, no. 6, pp. 302–308.
- Hawley, J. W., 1993, Overview of the geomorphic history of the Carlsbad area; in Love, D. W., Hawley, J. W., Kues, B. S., Adams, J. W., Austin, G. S., and Barker, J. M. (eds.), Carlsbad region, New Mexico and west Texas: New Mexico Geological Society, Guidebook 44, pp. 2–4.
- Hawley, J. W., Bachman, G. O., and Manley, K., 1976, Quaternary stratigraphy in the Basin and Range and Great Plains provinces, New Mexico and western Texas; in Mahaney, W. C. (ed.), Quaternary stratigraphy of North America: Dowen, Hutchinson and Ross, Inc., Stroudsburg, Pennsylvania, pp. 235–274.
- Hayes, P. T., 1964, Geology of the Guadalupe Mountains, New Mexico: U.S. Geological Survey, Professional Paper 446, 65 pp.
- Hill, C. A., 1987, Geology of Carlsbad Cavern and other caves in the Guadalupe Mountains, New Mexico and Texas: New Mexico Bureau of Mines and Mineral Resources, Bulletin 117, 150 pp.
- Kelley, V. C., 1971, Geology of the Pecos country, southeastern New Mexico: New Mexico Bureau of Mines and Mineral Resources, Memoir 24, 78 pp.
- Lambert, D., and the Diagram Group, 1988, The field guide to geology: Facts on File, Inc., New York, 256 pp.
- McLemore, V. T., 1989, Santa Rosa Lake State Park: New Mexico Geology, v. 11, no. 1, pp. 8–10.
- McLemore, V. T., 1996, Villanueva State Park: New Mexico Geology, v. 18, no. 2, pp. 38–41
- McLemore, V. T., 1999, Bottomless Lakes State Park: New Mexico Geology, v. 21, no. 2, pp. 51–55.
- McLemore, V. T., 2001, Living Desert Zoo and Gardens State Park: New Mexico Geology, v. 23, no. 2, pp. 42–50.
- Ward, R. F., Kendall, C. G. St. C., and Harris, P. M., 1986, Upper Permian (Guadalupian) facies and their association with hydrocarbons in the Permian Basin, west Texas and New Mexico: American Association of Petroleum Geologists Bulletin, v. 70, pp. 239–262.